Abstract
Adenylyl cyclase (AC) isoforms catalyze the synthesis of 3',5'-cyclic AMP from ATP. These isoforms are critically involved in the regulation of gene transcription, metabolism, and ion channel activity among others. Nitric oxide (NO) is a gaseous product whose synthesis from L-arginine is catalyzed by the enzyme NO synthase. It has been well established that NO activates the enzyme guanylyl cyclase, but little has been reported on the effects of NO on other important second messengers, such as AC. In the present study, the effects of sodium nitroprusside (SNP), a nitric oxide-releasing compound, on COS-7 cells transfected with plasmids containing AC types I, II, V and VI were evaluated. Total inhibition (~98.5%) of cAMP production was observed in COS-7 cells transfected with the AC I isoform and previously treated with SNP (10 mM) for 30 min, when stimulated with ionomycin. A high inhibition (~76%) of cAMP production was also observed in COS-7 cells transfected with the AC VI isoform and previously treated with SNP (10 mM) for 30 min, when stimulated with forskolin. No effect on cAMP production was observed in cells transfected with AC isoforms II and V.
Adenylyl cyclase; Nitric oxide; Sodium nitroprusside; Cyclic AMP; COS-7 cells; Signal transduction
Braz J Med Biol Res, February 2002, Volume 35(2) 145-151
Adenylyl cyclase types I and VI but not II and V are selectively inhibited by nitric oxide
J. Goldstein1,2, C. Silberstein1 and C. Ibarra1
1Laboratorio de Fisiopatogenia, Departamento de Fisiología, Facultad de Medicina, Universidad de Buenos Aires, Buenos Aires, Argentina
2Department of Neurobiology, The Weizmann Institute of Science, Rehovot, Israel
References
Correspondence and Footnotes Correspondence and Footnotes Correspondence and Footnotes
Adenylyl cyclase (AC) isoforms catalyze the synthesis of 3',5'-cyclic AMP from ATP. These isoforms are critically involved in the regulation of gene transcription, metabolism, and ion channel activity among others. Nitric oxide (NO) is a gaseous product whose synthesis from L-arginine is catalyzed by the enzyme NO synthase. It has been well established that NO activates the enzyme guanylyl cyclase, but little has been reported on the effects of NO on other important second messengers, such as AC. In the present study, the effects of sodium nitroprusside (SNP), a nitric oxide-releasing compound, on COS-7 cells transfected with plasmids containing AC types I, II, V and VI were evaluated. Total inhibition (~98.5%) of cAMP production was observed in COS-7 cells transfected with the AC I isoform and previously treated with SNP (10 mM) for 30 min, when stimulated with ionomycin. A high inhibition (~76%) of cAMP production was also observed in COS-7 cells transfected with the AC VI isoform and previously treated with SNP (10 mM) for 30 min, when stimulated with forskolin. No effect on cAMP production was observed in cells transfected with AC isoforms II and V.
Key words: Adenylyl cyclase, Nitric oxide, Sodium nitroprusside, Cyclic AMP, COS-7 cells, Signal transduction
Abstract
Introduction
Adenylyl cyclase (AC) (ATP pyrophosphate-lyase, cycling; EC 4.6.1.1) catalyzes the synthesis of 3',5'-cyclic AMP (cAMP) from ATP. Several mammalian AC isoforms have been cloned (1-3). These isoforms are involved critically in mammalian signal transduction pathways where they regulate gene transcription, metabolism, and ion channel activity (4,5). ACs are induced or inhibited by a system of three components associated with the plasma membrane: G protein-coupled receptors, stimulatory and inhibitory heterotrimeric G proteins, and the AC catalytic entity itself (6). AC types I and III are stimulated by Ca2+ and calmodulin (7), whereas types II and IV-VI are not (3,8). Furthermore, forskolin binds to the AC catalytic core (9,10) by joining the two domains of the core using a combination of hydrophobic and hydrogen-bonding interactions that are distributed equally between the two domains (11). It has been shown that all mammalian AC isoforms are activated by both forskolin and the GTP-bound a subunit of the stimulatory G protein Gs (12).
Nitric oxide (NO) is a gas whose synthesis is catalyzed by the enzyme NO synthase (NOS; EC 1.14.13.39). NOS activation is NADPH dependent by oxidation of the guanidine nitrogen of its substrate L-arginine in the presence of molecular oxygen and several other cofactors (FMN, FAD, tetrahydrobiopterin, heme moiety). Three NOS isoforms have been identified so far: NOS I (brain or neuronal NOS), NOS II (macrophage or hepatocyte NOS) and NOS III (endothelial NOS) (13). The diverse activities of NO include blood vessel relaxation and modulation of immune responses, pathogen killing, and inhibition of platelet aggregation and adhesion. Moreover, NO controls the activity of enzymes and ion channels, and serves as a neuromodulator and neurotransmitter in the central and peripheral nervous systems (14). There is also evidence that NO may be a physiological intracellular regulator of mitochondrial respiration and gene activity, and can interact with oxygen-derived radicals to produce other highly reactive substances (15,16). In addition, NO is involved in the development of tolerance to and withdrawal from morphine (17,18). Overproduction of NO in mammalian systems may contribute to cell damage or cell death (19). Recently, a number of new actions of NO have been proposed: among them protein modification by S-nitrosylation via the transfer of NO+ from nitrosothiols (20).
Although the actions of the enzymes AC and NOS in promoting two signal transduction pathways have been already characterized, little information is available about the relation between these two pathways. It has been well established that NO activates the second messenger guanylyl cyclase, but little has been reported on the effects of NO on other important second messengers such as AC. It has been previously demonstrated that the inhibition of the endogenous neuroblastoma cell line AC by NO is related to isoform type VI (21), but little information is available on the effects of NO on other mammalian AC isotypes, as in AC genes transfected to mammalian cultured cell lines.
In the present study we evaluated the effects of NO on the activity of mammalian AC isoforms I, II, V and VI in transfected mammalian cultured kidney COS-7 cells using the NO-releasing compound sodium nitroprusside (SNP) as measured by cAMP production.
Material and Methods
SNP was purchased from Alexis Biochemicals (San Diego, CA, USA). [3H-2]-Adenine (18.0 Ci/mmol) was from American Radiolabeled Chemicals (St. Louis, MO, USA), ionomycin and the phosphodiesterase inhibitors 1-methyl-3-isobutylxanthine (IBMX) and RO-20-1724 were from Calbiochem (La Jolla, CA, USA). Forskolin, cAMP, and thyroid-stimulating hormone (TSH) were from Sigma (St. Louis, MO, USA). Finally, tissue culture reagents were from Life Technologies (Gaithersburg, MD, USA).
Cell culture and transfection
COS-7 cells were cultured in Dulbecco's modified Eagle's medium (DMEM) supplemented with 5% fetal calf serum, 0.2 mg/ml G418 and 100 IU/ml penicillin, in a humidified atmosphere consisting of 5% CO2 and 95% air at 37ºC. Plasmids containing AC cDNAs (pXMD1-AC-I, pXMD1-AC-II, pXMD1-AC-V and pXMD1-AC-VI) have been described (22). Transient transfection of COS-7 cells was performed as described (22,23). Briefly, COS-7 cells on 10-cm plates were transfected using the DEAE-dextran chloroquine method with 2 µg/plate of one of the AC isozyme cDNAs. Twenty-four hours later, the cells from each 10-cm plate were trypsinized and recultured on a 24-well plate, and after an additional 24 h, cells were assayed for cAMP content (as a measure of AC activity) as described below. Transfection efficiency was normally in the range of 60-80%, as determined by staining for ß-galactosidase activity (24).
Sodium nitroprusside treatment and cAMP assay
The assay was performed in triplicate as described previously (22,25,26). Briefly, cells cultured on 24-well plates were incubated for 2 h with 0.25 ml/well of fresh growth medium containing 5 µCi/ml of [3H]-adenine; during the last 30 min SNP at the indicated concentrations and phosphodiesterase inhibitors (described below) were added to the incubation media, and then washed with 0.5 ml/well of DMEM containing 20 mM HEPES, pH 7.4, and 0.1 mg/ml BSA. This medium was replaced with 0.25 ml/well of DMEM containing 20 mM HEPES, pH 7.4, 0.1 mg/ml BSA, and the phosphodiesterase inhibitors IBMX (0.5 mM) and RO-20-1724 (0.5 mM). AC activity was stimulated in the presence or absence of the indicated concentrations of SNP by the addition of either 1 µM forskolin, for stimulation of AC V and VI, 1 µM ionomycin for stimulation of AC I, or 0.1 mM TSH for stimulation of AC II (in this last case, cells were transfected together with the TSH receptor). After 10 min of incubation at room temperature, the medium was removed and the reaction terminated by the addition of perchloric acid containing 0.1 mM unlabeled cAMP, followed by neutralization with KOH, and the amount of [3H]-cAMP was determined by a two-step column separation procedure, as previously described (23,27).
Statistical analysis
Results are reported as the mean ± SEM of triplicate determinations of a representative experiment. P<0.05 vs control was considered to be significant.
Four AC isozyme types were studied according to their properties and sequence homologies: AC I which is stimulated by Ca2+/calmodulin; AC V and AC VI, stimulated by forskolin, and AC II, stimulated by TSH (28,29), to determine whether SNP affected cAMP production by the different isoforms. For this purpose, the COS-7 cell system, which readily allows transient transfection of the desired AC isozymes, was employed.
Effects of sodium nitroprusside on the AC I isotype
Pretreatment of the transfected cells with increasing concentrations of SNP resulted in a distinct attenuation of cAMP production by AC isoform I (Figure 1A). A 38% attenuation of cAMP production was observed in the presence of 0.1 mM SNP, the NO donor. In the concentration range between 0.1 and 1 mM SNP, however, no significant difference was observed, while a strong attenuation of cAMP production was obtained in the presence of 10 mM SNP (Figure 1A).
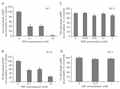
[View larger version of this image (44 K GIF file)]
Effects of sodium nitroprusside on the AC VI isotype
Similar results were obtained with the forskolin-stimulated AC VI isozyme. cAMP inhibition by NO donor SNP resulted in a distinct attenuation of cAMP production: 0.1 mM SNP resulted in 55% attenuation of cAMP production, while 24% attenuation was obtained using 10 mM SNP (Figure 1B).
Effects of sodium nitroprusside on the AC II and V isozymes
In contrast, no significant variations in cAMP production were detected in the TSH-stimulated AC II (Figure 1C) and forskolin-stimulated AC V isozymes (Figure 1D) when both isozymes were incubated with SNP under the same conditions as detailed above. Furthermore, in all the experiments performed, the basal accumulation of cAMP, a measure of cAMP generated in the absence of hormonal stimulation, was unaffected by treatment with SNP (data not shown).
Results
The present results suggest a selective inhibition by the NO donor SNP in the transfected AC isoforms. Both the AC VI and AC V isozymes are stimulated by the hormone forskolin, but only AC VI was inhibited by SNP. The results observed for the AC I and VI isotypes indicate that SNP inhibition occurs at the level of the enzyme, and thus is independent of the receptor/G protein-mediated pathway. The results also suggest that the inhibitory effect of SNP is independent of forskolin binding in these isoforms, resulting in a differential effect of SNP on the various AC isotypes. It is also interesting to mention that the inhibitory effect of SNP was on the AC I and AC VI isoforms, which are not closely related to each other either in terms of enzymatic stimulation or of genetic homology.
The complete coding sequence of AC type I is known, and there is some information concerning functional domains of the enzyme. An examination of the amino acid sequence of AC type I reveals several sequences which exhibit the general properties of calmodulin-binding domains. It has been reported that a synthetic peptide, corresponding to amino acids 495-522 from AC I, binds with high affinity and inhibits calmodulin stimulation of the enzyme, suggesting that this sequence may correspond to the calmodulin-binding domain of the enzyme (30). Furthermore, a point mutation within this domain abolished Ca2+ and calmodulin sensitivity of the enzyme (31). Interestingly, this sequence contains two cysteine residues, uncommon for calmodulin-binding domains. Pretreatment of recombinant COS-7 cells with SNP inhibits catalytic activity of the transfected AC I isozyme (Figure 1A). One possible explanation for this inhibition could be the oxidation of sulfhydryl groups of neighboring cysteines in the calmodulin-binding domain of the enzyme. The site of the NO effect on the enzyme is intriguing; since the basal enzyme activity of the different AC isozymes did not vary, it is unlikely that NO action could be targeted to the catalytic site of the enzyme. In agreement with these results, inhibition of the forskolin-stimulated AC VI isotype by pretreatment with SNP of transfected COS-7 cells was also detected (Figure 1B).
The mechanism by which we propose selective NO blockage of the AC I and AC VI isoforms is in agreement with recent reports suggesting that the conformation of the enzymes AC I and AC VI determines their sensitivity (21,32,33). The basal activities of type I and VI recombinant enzymes are not altered by NO, suggesting that, in their basal states, these isoforms share a conformation that precludes susceptibility to NO. On this basis, we speculate that this inhibition is due to a change in Vmax, with no change in the Km of the substrate. Future experiments will be performed in order to analyze the kinetic effects of NO on cAMP production in stimulated, transfected COS-7 cells incubated with SNP and assayed at increasing [3H]-adenine concentrations.
Although it has been reported that NO did not inhibit cAMP production by the AC I isotype (32), those experiments were performed on insect cell membranes. In other previous studies (21) it was observed that SNP inhibited endogenous AC type VI activation in cultured neuroblastoma N18TG2 cells.
The present study provides evidence for the selective inhibition of cAMP production by AC enzyme types I and VI, but not types II and V, in AC-transfected cultured mammalian cell lines treated with the NO donor SNP. It is interesting to observe that both enzymes, AC I and AC VI, share a conformation that precludes any susceptibility to NO (33), suggesting that SNP might be nitrosylating two cysteine residues, thus modifying their catalytic activity. A possible means of testing this hypothesis is to induce a mutation of the residues in genetically modified AC isoforms. These results would be interesting since differential expressions of AC isotypes occur in different organs and tissues in mammals. All AC isoforms appear to be expressed in the brain, apparently in region-specific patterns; the differential expression of AC isoforms in the central nervous system has been particularly well studied. In addition, there is a predominant expression of AC I in area CA1 of the hippocampus (34). NO plays a primary role in learning and memory in the hippocampus; advanced studies on the regulation of AC by NO would shed more light on the understanding of this field. Although the inhibition observed in cultured COS-7 cells transfected with plasmids containing AC I and AC VI probably would not reflect the same results performed in vivo due to numerous active pathways interacting in concert, as is the case for specific regions of the brain, transfection experiments with engineered cyclase isoforms should provide powerful new tools to determine in vivo how a particular regulator controls a particular AC isoform in the context of many simultaneous active pathways. Recently, it has been reported that NO inhibits AC activity in brain striatal membranes (35), in agreement with the results obtained here for cultured cells. Hudson et al. (35) found that the AC I isoform was present in neurons of the striatum, together with the AC V and AC VI isoforms. Thus NO could modulate the dopaminergic regulation of cAMP along with the effects of Ca2+, modifying intracellular events in striatal neurons (36). NO has been implicated in the destruction of dopaminergic neurons and their projecting nerve fibers in the striatum that characterizes Parkinson's disease (36). Therefore, vectors containing AC isoforms transfected to striatal neurons would provide an interesting approach for a treatment aimed at preventing Parkinson's disease.
Discussion
Acknowledgments
We wish to thank Prof. Dr. T. Pfeuffer, Prof. Dr. A. Gilman, and Prof. Dr. Jack Krupinski for providing adenylyl cyclase expression vectors, and Prof. Dr. Zvi Vogel for suggestions and advice.
Address for correspondence: J. Goldstein, Laboratorio de Fisiopatogenia, Departamento de Fisiología, Facultad de Medicina, Universidad de Buenos Aires, Paraguay 2155, Piso 7, Buenos Aires 1121, Argentina. Fax: +54-11-4508-3653. E-mail: jogol@fmed.uba.ar
Research supported by the grant PIP 4549/96 (CONICET). Received March 5, 2001. Accepted November 6, 2001.
- 1. Krupinski J, Coussen F, Bakalyar HA, Tang WJ, Feinstein PG, Orth K, Slaughter C, Reed RR & Gilman AG (1989). Adenylyl cyclase amino acid sequence: possible channel- or transporter-like structure. Science, 244: 1558-1564.
- 2. Bakalyar HA & Reed RR (1990). Identification of a specialized adenylyl cyclase that may mediate odorant detection. Science, 250: 1403-1406.
- 3. Gao B & Gilman AG (1991). Cloning and expression of a widely distributed (type IV) adenylyl cyclase. Proceedings of the National Academy of Sciences, USA, 88: 10178-10182.
- 4. Krupinski J (1991). The adenylyl cyclase family. Molecular and Cellular Biochemistry, 104: 73-79.
- 5. Tang WJ, Krupinski J & Gilman AG (1991). Expression and characterization of calmodulin-activated (type I) adenylyl cyclase. Journal of Biological Chemistry, 266: 8595-8603.
- 6. Taussig R & Gilman AG (1995). Mammalian membrane-bound adenylyl cyclases. Journal of Biological Chemistry, 270: 1-4.
- 7. Choi EJ, Wong ST, Hinds TR & Storm DR (1992). Calcium and muscarinic agonists stimulation of type I adenylyl cyclase in whole cells. Journal of Biological Chemistry, 267: 12440-12442.
- 8. Feinstein PG, Schrader KA, Bakalyar HA, Tang WJ, Krupinski J, Gilman AG & Reed RR (1991). Molecular cloning and characterization of a Ca2+/calmodulin-insensitive adenylyl cyclase from rat brain. Proceedings of the National Academy of Sciences, USA, 88: 10173-10177.
- 9. Tesmer JJG, Sunahara RK, Gilman AG & Sprang SR (1997). Crystal structure of the catalytic domains of adenylyl cyclase in a complex with GsaşGTPgS. Science, 278: 1907-1916.
- 10. Hurley JH (1999). Structure, mechanism, and regulation of mammalian adenylyl cyclase. Journal of Biological Chemistry, 274: 7599-7602.
- 11. Zhang G, Liu Y, Ruoho AE & Hurley JH (1997). Structure of the adenylyl cyclase catalytic core. Nature, 386: 247-253.
- 12. Pfeuffer T & Metzger H (1982). 7-O-Hemisuccinyl-deacetyl forskolin-Sepharose: a novel affinity support for purification of adenylate cyclase. FEBS Letters, 146: 369-375.
- 13. Griffith OW & Stuehr DJ (1995). Nitric oxide synthases: Properties and catalytic mechanism. Annual Review of Physiology, 57: 707-736.
- 14. Wolf G (1997). Nitric oxide and nitric oxide synthase: biology, pathology, localization. Histology and Histopathology, 12: 251-261.
- 15. Brown GC (1995). Nitric oxide regulates mitochondrial respiration and cell functions by inhibiting cytochrome oxidase. FEBS Letters, 369: 136-139.
- 16. Moncada S, Feelisch M, Busse R & Higgs EA (Editors) (1994). The Biology of Nitric Oxide: Enzymology, Biochemistry and Immunology Portland Press, London, UK.
- 17. Bhargava HN (1995). Attenuation of tolerance to, and physical dependence on, morphine in the rat by inhibition of nitric oxide synthase. General Pharmacology, 26: 1049-1053.
- 18. Vaupel DB, Kimes AS & London ED (1995). Comparison of 7-nitroindazole with other nitric oxide synthase inhibitors as attenuators of opioid withdrawal. Psycopharmacology (Berlin), 118: 361-368.
- 19. Gross SS & Wolin MS (1995). Nitric oxide synthases: pathophysiological mechanisms. Annual Review of Physiology, 57: 737-769.
- 20. Stamler JS (1994). Redox signaling characterization of 125I-labeled secretin binding and association with adenylyl cyclase. Journal of Neurochemistry, 42: 1145-1152.
- 21. McVey M, Hill J, Howlett A & Klein C (1999). Adenylyl cyclase, a coincidence detector for nitric oxide. Journal of Biological Chemistry, 274: 18887-18892.
- 22. Avidor-Reiss T, Nevo I, Saya D, Bayewitch M & Vogel Z (1997). Opiate-induced adenylyl cyclase superactivation is isozyme-specific. Journal of Biological Chemistry, 272: 5040-5047.
- 23. Avidor-Reiss T, Nevo I, Levy R, Pfeuffer T & Vogel Z (1996). Chronic opioid treatment induces adenylyl cyclase V superactivation. Involvement of Gßg Journal of Biological Chemistry, 271: 21309-21315.
- 24. Lim K & Chae CB (1989). A simple assay for DNA transfection by incubation of the cells in culture dishes with substrates for betagalactosidase. Biotechniques, 7: 576-579.
- 25. Avidor-Reiss T, Bayewitch M, Levy R, Matus-Leibovitch N, Nevo I & Vogel Z (1995). Adenylyl cyclase supersensitization in ľ-opioid receptor-transfected Chinese hamster ovary cells following chronic opioid treatment. Journal of Biological Chemistry, 270: 29732-29738.
- 26. Nevo I, Avidor-Reiss T, Levy R, Bayewitch M, Heldman E & Vogel Z (1998). Regulation of adenylyl cyclase isozymes upon acute and chronic activation of inhibitory receptors. Molecular Pharmacology, 54: 419-426.
- 27. Salomon Y (1991). Cellular responsiveness to hormones and neurotransmitters: conversion of [3H]cAMP in cell monolayers, cell suspensions and tissue slices. Methods in Enzymology, 195: 22-28.
- 28. Choi EJ, Xia Z, Villacres EC & Storm DR (1993). The regulatory diversity of the mammalian adenylyl cyclases. Current Opinion in Cell Biology, 5: 269-273.
- 29. Bayewitch ML, Avidor-Reiss T, Levy R, Pfeuffer T, Nevo I, Simonds WF & Vogel Z (1998). Inhibition of adenylyl cyclase isoforms V and VI by various Gßg subunits. FASEB Journal, 12: 1019-1025.
- 30. Vorherr T, Knopfel L, Hofmann F, Mollner S, Pfeuffer T & Carafoli E (1993). The calmodulin binding domain of nitric oxide synthase and adenylyl cyclase. Biochemistry, 32: 6081-6088.
- 31. Wu Z, Wong ST & Storm DR (1993). Modification of the calcium sensitivity of the type I adenylyl cyclase by mutagenesis of its calmodulin binding domain. Journal of Biological Chemistry, 268: 23766-23768.
- 32. Hill J, Howlett A & Klein C (2000). Nitric oxide selectively inhibits adenylyl cyclase isoforms 5 and 6. Cellular Signalling, 12: 233-237.
- 33. Duhe RJ, Nielsen MD, Dittman AH, Villacres EC, Choi E & Storm DR (1994). Oxidation of critical cysteine residues of type I adenylyl cyclase by o-Iodosobenzoate or nitric oxide reversibly inhibits stimulation by calcium and calmodulin. Journal of Biological Chemistry, 269: 7290-7296.
- 34. Chetkovich DM, Gray R, Johnston D & Sweatt JD (1991). N-Methyl-D-aspartate receptor activation increases cAMP levels and voltage-gated Ca2+ channel activity in area CA1 of hippocampus. Proceedings of the National Academy of Sciences, USA, 88: 6467-6471.
- 35. Hudson TY, Corbett JA, Howlett AC & Klein C (2001). Nitric oxide regulates adenylyl cyclase activity in rat striatal membranes. Journal of Neurochemistry, 77: 1279-1284.
- 36. Calabresi P, Centonze D, Gubellini P, Marfia GA, Pisani A, Sancessario G & Bernardi G (2000). Synaptic transmission in the striatum: from plasticity to neurodegeneration. Progress in Neurobiology, 61: 231-265.
Correspondence and Footnotes
Publication Dates
-
Publication in this collection
08 Feb 2002 -
Date of issue
Feb 2002
History
-
Received
05 Mar 2001 -
Accepted
06 Nov 2001