Abstracts
Selected schemes conceived for providing reliable and computationally efficient estimates of the absorption of solar radiation by the atmospheric water vapor are discussed. Special attention is devoted to the scheme proposed by Lacis & Hansen, which is included in radiative codes of atmospheric models running at Brazilian centers of research and numerical weather forecasting. Additional schemes, proposed from relatively modern estimates of the effects due to the water vapor absorption, are also included in the analysis. In the goal of assessing systematic errors associated to these schemes, comparisons are performed with benchmark results available in the literature which have been obtained through careful line-by-line integration throughout the solar spectrum. Modern schemes allow more atmospheric absorption, and therefore less downward shortwave irradiance at the ground, when compared with schemes implicitly adopted at present in Brazilian centers.
Solar radiation; Water vapor; Radiative transfer; Atmospheric modeling; Line-by-line integration; Radiative codes
Este artigo analisa diversos aspectos relacionados à avaliação dos efeitos da absorção de radiação solar pelo vapor d'água na atmosfera, particularmente quando efetuada através de esquemas (parametrizações) que sejam acurados e com baixo custo computacional. Atenção especial é dedicada ao esquema proposto por Lacis & Hansen, dado que ele encontra-se incluído em códigos radiativos de modelos atmosféricos utilizados em diversas instituições brasileiras. As análises incluem ainda outros esquemas, propostos a partir de estimativas relativamente modernas dos efeitos devidos à absorção de radiação solar pelo vapor d'água. No intuito de se quantificar erros sistemáticos associados a estes esquemas, comparações são efetuadas frente a resultados disponíveis na literatura e obtidos empregando-se códigos radiativos capazes de levar em conta o impacto isolado de cada linha de absorção. Esquemas de desenvolvimento recente proporcionam mais absorção atmosférica, e portanto menores valores para a irradiância solar incidente à superfície do solo, quando comparados com aqueles esquemas que têm sido adotados implicitamente em modelos atmosféricos empregados em instituições brasileiras.
Radiação solar; Vapor d'água; Transferência radiativa; Modelagem atmosférica; Integração linha-a-linha; Códigos radiativos
Absorption of solar radiation by water vapor in the atmosphere. Part I: a comparison between selected parameterizations and reference results.
A. Plana-Fattori1, E. P. de Souza1,2 & J. C. S. Chagas3
1Departamento de Ciências Atmosféricas
Instituto Astronômico e Geofísico - Universidade de São Paulo
Rua do Matão, 1226 - Cidade Universitária - CEP 05508-900 - São Paulo - SP
Fone: (011)818-4712/4713 - Fax: (011)818-4714
e-mail artemio@model.iag.usp.br
2on leave from:
Departamento de Ciências Atmosféricas
Centro de Ciência e Tecnologia - Universidade Federal da Paraíba
3Centro de Previsão de Tempo e Estudos Climáticos
Instituto Nacional de Pesquisas Espaciais
Rodovia Presidente Dutra, Km 40 - CEP 12630-000 - Cachoeira Paulista - SP
Selected schemes conceived for providing reliable and computationally efficient estimates of the absorption of solar radiation by the atmospheric water vapor are discussed. Special attention is devoted to the scheme proposed by Lacis & Hansen, which is included in radiative codes of atmospheric models running at Brazilian centers of research and numerical weather forecasting. Additional schemes, proposed from relatively modern estimates of the effects due to the water vapor absorption, are also included in the analysis. In the goal of assessing systematic errors associated to these schemes, comparisons are performed with benchmark results available in the literature which have been obtained through careful line-by-line integration throughout the solar spectrum. Modern schemes allow more atmospheric absorption, and therefore less downward shortwave irradiance at the ground, when compared with schemes implicitly adopted at present in Brazilian centers.
Key words: Solar radiation; Water vapor; Radiative transfer; Atmospheric modeling; Line-by-line integration; Radiative codes.
Absorção de radiação solar por vapor d'água na atmosfera. Parte I: uma comparação entre parametrizações e resultados de referência - Este artigo analisa diversos aspectos relacionados à avaliação dos efeitos da absorção de radiação solar pelo vapor d'água na atmosfera, particularmente quando efetuada através de esquemas (parametrizações) que sejam acurados e com baixo custo computacional. Atenção especial é dedicada ao esquema proposto por Lacis & Hansen, dado que ele encontra-se incluído em códigos radiativos de modelos atmosféricos utilizados em diversas instituições brasileiras. As análises incluem ainda outros esquemas, propostos a partir de estimativas relativamente modernas dos efeitos devidos à absorção de radiação solar pelo vapor d'água. No intuito de se quantificar erros sistemáticos associados a estes esquemas, comparações são efetuadas frente a resultados disponíveis na literatura e obtidos empregando-se códigos radiativos capazes de levar em conta o impacto isolado de cada linha de absorção. Esquemas de desenvolvimento recente proporcionam mais absorção atmosférica, e portanto menores valores para a irradiância solar incidente à superfície do solo, quando comparados com aqueles esquemas que têm sido adotados implicitamente em modelos atmosféricos empregados em instituições brasileiras.
Palavras-chave: Radiação solar; Vapor d'água; Transferência radiativa; Modelagem atmosférica; Integração linha-a-linha; Códigos radiativos.
INTRODUCTION
General circulation models employed for climate modeling and numerical weather forecasting require accurate estimates of the absorption of radiation by atmospheric water vapor. As the explicit formulation of the problem is too complicated for general circulation modeling purposes, these estimates are based upon computationally efficient schemes designed for obtaining the mean value of the water vapor absorptance over a broad fraction of the solar spectrum as a function of the water vapor mass content. The simplicity of such broadband absorptances schemes is illusory, once they allow only the combined effects of thousands of absorption lines, throughout a beam path with inhomogeneous distributions of water vapor concentration, pressure and temperature. An overview on the subject may be found in some textbooks (e.g., Liou, 1992, pp. 26-47).
In this article, the reliability of selected schemes for obtaining water vapor broadband absorptances in atmospheric models is assessed by comparison with results achieved by other authors through reference methods. Attention is given to the schemes proposed by Lacis & Hansen (1974) because it is included, sometimes with modifications, in radiative codes of atmospheric models running at Brazilian centers of research and numerical weather forecasting. This is the case of the radiative code proposed by Davies (1982), fully described by Chagas & Tarasova (1996), which runs daily at the global model of the "Centro de Previsão do Tempo e Estudos Climáticos" (Center for Weather Forecast and Climate Studies, CPTEC/INPE, Cachoeira Paulista, SP), a version of the general circulation model of the Center for Ocean-Land-Atmosphere Studies (COLA/IGES, USA; see Sato et al., 1989, for an overview). This is also the case of the radiative code of Chen & Cotton (1983) included in the regional atmospheric model RAMS (Pielke et al., 1992). The scheme of Lacis & Hansen (1974) is considered yet in some general circulation models for obtaining either climate projections (see Gates, 1992, for a compilation in the scope of the program AMIP) or numerical weather forecasts (e.g.: Black, 1994; Hardiker, 1997). Special attention is given also in this study to recent schemes which could replace the one proposed by Lacis & Hansen (1974) in atmospheric models.
The companion article (Souza et al., 1998, this issue) deals with the influence of the scheme employed for obtaining water vapor broadband absorptances on several meteorological fields. Such an influence is assessed from numerical simulations made with the CPTEC/COLA's general circulation model. Taken together, these two articles could indicate (i) the magnitude of systematic errors due to the water vapor absorption calculations, and (ii) the possibility of reducing them by replacing current schemes with more recent ones.
OVERVIEW ON THE SUBJECT
Broadband absorptances ao (due to water vapor, carbon dioxide, etc.) are defined from respective monochromatic a(n) values by means of weighted-averages, taking into account the spectral distribution Eon(n) associated to the solar constant Eo :
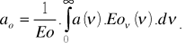
(1)
As the contribution of the water vapor to the molecular scattering is negligible in the terrestrial atmosphere, water vapor absorptances a(n) can be assumed complementary of the respective beam transmittances t(n), allowing:
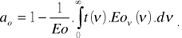
(2)
Water vapor transmittances for a slant downwelling path between the pressure level p'=p and the top of the atmosphere (p'» 0) can be written in the form:

(3)
where M(mo) is the optical air mass, mo is the cosine of the Sun zenith angle, g is the acceleration due to the gravity, T(p') and q(p') are respectively the air temperature and the specific humidity (kg.kg-1) at the level p', and k[v,p',T(p')] is the mass absorption coefficient (m2.kg-1). For a wavenumber n, such coefficient results from the contribution of J absorption lines centered at wavenumbers noj (Liou, 1992, p.44):

(4)
being that each one corresponding to an integrated line intensity Sj[T(p')] and to a line shape factor fj[n,noj,p',T( p')]. Recent compilations of absorption line parameters (e.g., Rothman et al., 1992) contain information about more than 48500 transitions for the water vapor between v=0 and v=23000cm-1.
The accurate integration of the right-hand side of Eq.(2) for a given atmospheric profile (the so-called line-by-line approach) requires a very fine spectral resolution, around 0.01cm-1 for the water vapor absorption (Liou, 1992, p.44). It represents a formidable time consuming task, even with the availability of a supercomputer. This approach is adopted mainly for obtaining benchmark results, which have been many times employed for assessing the reliability of schemes included in radiative codes conceived to numerical weather forecasting and climate modeling.
Most schemes running at present in atmospheric models have been proposed in the context of two methods, the k-distribution and the exponential sum fitting of transmissions (ESFT). Both approaches enable a consistent merge between multiple scattering and molecular absorption calculations (Stephens, 1984). Following both methods, broadband absorptances can be obtained through relationships like:

(5)
where U is the path-integrated water vapor content. In the k-distribution method, P(kn) and kn indicate discrete probability distribution values and absorption coefficients. The fundamentals of this method as well as the rationale for obtaining P(kn) and kn from line-by-line calculations may be found in recent textbooks (e.g., Liou, 1992, pp.72-77). The ESFT method enables broadband absorptances also by applying Eq.(5), but P(kn) and kn are obtained by minimizing an error function to approximate the true values of 1-ao (e.g., Armbruster & Fischer, 1996).
Tab. 1 displays P(kn) and kn corresponding to the schemes compared in this study (see Section 4). As the accuracy of the Eq.(5) increases with N (see Fig. 1 of Chou & Lee, 1996), these schemes would in principle furnish different results even to the case where they have been obtained from a same set of previous computations. The schemes proposed by Lacis & Hansen (1974) and by Davies (1982) were developed through the ESFT method from broadband absorptance calculations performed by Yamamoto (1962), who employed overall absorption data (see Section 3). The scheme proposed by Briegleb (1992) includes kn values obtained by Lacis & Hansen (1974), and P(kn) values adjusted for giving satisfactory agreement with line-by-line calculations. Ramaswamy & Freidenreich (1992) also applied the ESFT method, but fitting the differences between results from line-by-line calculations and those obtained with the Lacis & Hansen scheme. The scheme of Chou & Lee (1996) was derived through the k-distribution method from line-by-line calculations.
Radiative heating rates for ICRCCM cases 3 and 4 (see Tab.2), resulting from the application of selected schemes for obtaining water vapor broadband absorptances (LH: Lacis & Hansen, 1974; D: Davies, 1982; RF: Ramaswamy & Freidenreich, 1992; Briegleb, 1992; and CL: Chou & Lee, 1996).
Figura 1 - Taxas de aquecimento radiativo para os casos 3 e 4 do programa ICRCCM (ver Tab.2), obtidas aplicando-se diferentes esquemas para avaliação da absortância solar devida ao vapor d'água (LH: Lacis & Hansen, 1974; D: Davies, 1982; RF: Ramaswamy & Freidenreich, 1992; Briegleb, 1992; e CL: Chou & Lee, 1996).
Discrete probability function values P(kn) and absorption coefficients knrespective to selected schemes proposed for obtaining broadband absorptances. knvalues are expressed in (kg/m2)-1. P(kn) values corresponding to the scheme of Briegleb (1992) result from the product of the respective p(H2O) with the factor solfrc, fraction of solar irradiance in the interval 0.7-5.0 mm (see Tab.1b of that author). Last line indicates the spectral range over which water vapor absorption was taken into account in the development of every scheme.
Tabela 1 - Valores da função discreta de probabilidade P(kn) e coeficientes de absorção knpara diferentes esquemas propostos para avaliação da absortância solar devida ao vapor d'água. Valores de knsão expressos em (kg/m2)-1. Valores de P(kn) para o esquema de Briegleb (1992) foi obtido multiplicando-se o respectivo valor p(H2O) pelo fator solfrc, fração da irradiância solar contida no intervalo 0,7-5,0 mm (ver Tab.1b daquele autor). A última linha indica o domínio ao longo do qual a absorção pelo vapor d'água foi levada em conta no desenvolvimento de cada esquema.
Realistic atmospheres are characterized by variations of air temperature, pressure and absorber amount along the optical path. A classical approach for evaluating water vapor absorption in such conditions deals with the so-called scaling approximation, which involves the adoption of an equivalent optical path, at fixed pressure and temperature, which replaces the real one. In other words, it could be possible to identify a "reference level" in the atmosphere, characterized by p'=pr and T(p')=Tr , whose adoption would allow the same transmittance resulting from the realistic profile. It is usual to assume in such context that the ratio between the mass absorption coefficient k[v,p',T(p')] and its respective value at (pr,Tr) is a function which does not exhibit any spectral dependence,

(6)
allowing monochromatic transmittances given by:

(7)

(8)
where We(p) is the equivalent (or pressure-temperature-weighted) vertically integrated water vapor content. Different scaling functions F[p',T(p'),pr,Tr ] have been proposed, allowing distinct We(p) values for a given atmospheric profile. Most schemes were developed by adopting

(9)
with pr=1013hPa and Tr=273K. According to Lacis & Hansen (1974), "there is little theoretical justification for using [our Eq.(9)] for the entire spectrum of water vapor bands, but it is probably better than applying no pressure and temperature correction at all". Typical values of n and m for the water vapor in the shortwave domain range from 0.9 to 1 and from 0.45 to 0.5, respectively (Stephens, 1984). The influence of n on broadband absorptances have been analyzed by some authors (Lacis & Hansen, 1974; Wang, 1976). According to Stephens (1984), the uncertainties associated with the application of Eq.(9) and the subsequent choice of n are smaller than those associated with uncertainties in both the absorption data and the Eon(n) spectrum.
Lacis & Hansen (1974), Davies (1982), and Ramaswamy & Freidenreich (1992) employed n=1 and m=0.5 in their schemes for obtaining ao estimates. In order to provide more realistic stratospheric heating rates, Briegleb (1992) employed a modified pressure-weighted path based upon the scaling function given by:

(10)
being mo the cosine of the Sun zenith angle and d=0.0017 an empirical factor determined by trial and error. Following an alternative point of view, Chou & Lee (1996) adopted the scaling function

(11)
with pr=300hPa, Tr=240K, and n=0.8 . The influence of somewhat different values for pr , Tr , and n in Eq.(11) on water vapor broadband absorptances was discussed by Chou (1986). The choice of the reference pressure level in the upper troposphere instead of at the mean sea level was justified by Chou & Arking (1981) from a careful analysis involving (a) the relative importance of line centers and line wings to the absorption at a given level and (b) the basic features of the water vapor vertical distribution.
BROADBAND ABSORPTANCES FROM MEASUREMENTS OF OVERALL ABSORPTION
Broadband absorptances can also be derived from measurements of the overall absorption due to the water vapor throughout a series of well-defined spectral intervals. Supposing that significative absorption takes place only at a finite number I of spectral intervals Dni ,then:

(12)
Assuming that molecular absorptances exhibit more important spectral dependence than that related to the solar irradiance, it is possible to define an average value Eon(Dni) for each interval Dni :

(13)
which can be evaluated, in a first-order approximation, by averaging Eon(n) over the respective interval. Equation (13) allows the identification of the integrals A(Dni ), which can be interpreted in terms of the total absorption verified throughout the spectral interval Dni or, alternatively, in terms of the equivalent band width of the same interval (Howard et al., 1956a). Thus, broadband absorptances can be written in the form:

(14)
being (Dni ) an "average" absorptance given by:

(15)
Yamamoto (1962), among others, followed such rationale to evaluate water vapor broadband absorptances, taking into account the most important absorption bands situated in the solar spectrum. He employed previous results obtained from two experimental programs conducted in the past, namely: (a) the curves relating average absorptances (Dni ) with the atmospheric water vapor content, resulting from the pioneer work of F. E. Fowle in the beginning of this century (Fowle, 1915), and (b) the analytical expressions describing total absorptions A(Dni ) which resume the measurements performed in laboratory by J. N. Howard, D. E. Burch and D. Williams in the fifties (Howard et al., 1956a, 1956b).
Major results from the pioneer work of Fowle (1915) were summarized by himself in a figure which shows a family of curves relating water vapor content values with atmospheric absorptances averaged over narrow intervals centered around 0.72- , 0.8- , 0.94- , 1.1- , 1.38- , and 1.87-mm. Such results were reviewed and applied by McDonald (1960), who concluded also (a) that Fowle's curves indicate average absorptances intrinsically reduced to sea level pressures, allowing computations of solar radiation absorption which depends only on the knowledge of the atmospheric pressure-weighted water vapor content, and (b) that the contribution of the weak 0.72- and 0.8-mm bands on the broadband value ao cannot be neglected, yet the respective values of the solar spectral irradiance are relatively high. The latter point was confirmed by Kratz & Cess (1985) from more recent molecular line data.
Howard et al. (1956a, 1956b) studied total absorptions associated to several water vapor bands from measurements performed under controlled conditions in laboratory proposing also first-order analytical descriptions for A(Dni). Following those authors, the total absorption associated to a certain water vapor band can be alternately evaluated by one of two expressions,

(16)

(17)
depending on values assumed by the mass content U (g/cm2) of the absorbing gas, by its partial pressure pa (mm Hg), and by the pressure pna (mm Hg) of the non-absorbing gaseous mixing. Parameters ci , ki , Ci , Di , and Ki are empirically-derived constants for each band. The two expressions correspond respectively to the cases A(Dni) < Ac(Dni) (weak-band fit) and A(Dni) > Ac(Dni) (strong-band fit), where Ac(Dni) denotes transition or critical values. These latter do not have a physical meaning, as stressed by Howard et al. (1956b): "... the selection of the transition value is somewhat arbitrary; in its immediate vicinity neither [our Eq.(16)] nor [our Eq.(17)] applies and an interpolation function must be used". Water vapor effects were investigated only for the bands centered around 0.94- , 1.1- , 1.38- , 1.87- , 2.7- , 3.2- , and 6.3-mm. Those authors claimed an agreement within 3 % between observed and estimated total absorptions by using Eqs.(16) and (17), with the exception of the 0.94-mm band. Howard et al. (1956b) recognized that other expressions besides Eqs.(16) and (17) may exist, which could be better justified from the theoretical point of view.
The calculations performed by Yamamoto (1962) represented one of the first efforts to merge results from the careful laboratory measurements made by Howard et al. (1956a, 1956b) concerning the most important water vapor bands in the solar spectrum, with those derived from Fowle's work and associated to the non-negligible 0.72- and 0.8-mm bands. Unfortunately, Yamamoto (1962) was somewhat confusing in explaining the framework of his approach. Results provided by Fowle (1915) were employed only for calculations corresponding to the 0.72- and 0.8-mm bands, under water vapor content values below 20 kg/m2 ; above such limit, estimates were inferred by analogy with absorptances given by Howard et al. (1956b) to the 1.1-mm band. Results from these latter authors were directly applied to the other bands. Figure 1 of Yamamoto (1962) shows a satisfactory agreement between Howard et al.'s and Fowle's data, if the latter are applied as indicated by McDonald (1960).
Lacis & Hansen (1974) proposed a scheme like Eq.(5) for obtaining water vapor broadband absorptances, with N=8 (see Tab.1). This expression was obtained by fitting the broadband absorptances displayed as curve 3 in Fig.1 of Yamamoto (1962). A similar scheme was proposed by Davies (1982), with N=5 (see Tab.1), from the same calculations. Both schemes allow ao estimates which include, intrinsically, limitations of the contributions from Howard et al. (1956a, 1956b) and from Fowle (1915), as well as restrictions concerning the assumptions accepted by Yamamoto (1962).
The reliability of the scheme proposed by Lacis & Hansen (1974) have been discussed by several authors (Stephens, 1984; Kratz & Cess, 1985; Chou, 1986; Ramaswamy & Freidenreich, 1992). Following those discussions, inaccurate estimates for ao could be obtained for relatively dry atmospheric layers. This would be related either to the limits of applicability of the fit proposed by Lacis & Hansen (1974) or to the unsuitability of the extrapolation criteria adopted by Yamamoto (1962) to estimate absorptances beyond experimental conditions. Nevertheless, two other aspects could in principle contribute to decrease the reliability of that parameterization.
First of all, the applicability of the results shown by Fowle (1915) to the evaluation of heating rates is a matter of controversy. As noted by Roach (1961): "... Fowle's experimental methods were sound but the reliability of his data is limited by the equipment and small theoretical knowledge then available: for instance, he did not study the effects of changing pressure and temperature on absorption, so that while his measurements of total absorption as a function of water vapour are approximately correct, they are inadequate for estimating the rate of change (1 1 Italic from Roach's article. ) of absorption with optical path which is directly related to the heating rate''. It seems adequate, following such reasoning, that any contributions resulting from Fowle's work should be employed only for total absorption calculations, and no longer for the computation of radiative heating rates.
Further, the reliability of the results obtained by Howard et al. (1956a, 1956b) is also a matter of controversy. Yamanouchi & Tanaka (1985), after conducting a somewhat similar experimental program, observed important discrepancies between their own total absorptions A(Dni) and those reported by Howard et al. (1956b) to the bands centered around 0.94- , 1.1- , and 1.38-mm. Yamanouchi & Tanaka (1985) attributed such discrepancies mainly to an inadequate treatment of the pressure dependence by those authors in their empirical relationships and to the influence of systematic experimental errors. As consequence of such discrepancies, water vapor broadband absorptances resulting from Yamamoto's calculations are smaller than those derived from Yamanouchi & Tanaka (1985) measurements by about 1 % in absolute value or 10 % in relative value.
Thus, it seems clear that the accuracy of water vapor broadband absorptances obtained through schemes based upon calculations performed by Yamamoto (1962) may be smaller than that usually accepted. This is the case of water vapor absorption estimates made by using the scheme of Lacis & Hansen (1974) employed in the regional atmospheric model RAMS, as well as those made through the scheme proposed by Davies (1982) and incorporated in the general circulation model running at the CPTEC/INPE.
SOME COMPARISONS
The accuracy of selected schemes conceived for obtaining water vapor broadband absorptances is discussed below after some comparisons with benchmark results. These latter have been obtained through careful line-by-line integration throughout a large fraction of the solar spectrum, following procedures described in some textbooks (e.g.: Liou, 1992, pp.26-47). Such benchmark results have been provided by different authors in the scope of a series of efforts devoted since the mid-eighties to compare radiative codes (program ICRCCM ¾ Ellingson & Fouquart (1991)).
All comparisons discussed here are performed under defined conditions for atmospheric profiles, Sun position and ground reflection (see Tab.2). These conditions represent the first six purely absorbing test cases employed for the shortwave computations of the ICRCCM program (Fouquart et al., 1991). For these six cases, shortwave radiative transfer is assumed to depend only on Sun position, on water vapor absorption, and on ground reflection of the direct beam.
Tab. 3 compares benchmark results published in recent years for a same ICRCCM shortwave case, displaying the respective spectral range over which the absorption by water vapor was taken into account, as well as the compilation which was adopted for absorption line parameters. The total atmospheric absorption is defined as (Fouquart et al., 1991):
Total atmospheric absorption ABS due to the water vapor for ICRCCM shortwave case 1 (see Tab.2), obtained through different line-by-line radiative codes. Compilations AFGL 1982, HITRAN 1986 and HITRAN 1992 have been described successively by Rothman et al. (1983, 1987, 1992).
Tabela 3 - Absorção atmosférica total ABS devida ao vapor d'água para o caso 1 do programa ICRCCM (ver Tab.2), obtida aplicando-se diferentes códigos radiativos capazes de levar em conta o impacto isolado de cada linha de absorção. As bases de dados espectroscópicos AFGL 1982, HITRAN 1986 e HITRAN 1992 foram descritas por Rothman et al. (1983, 1987, 1992).

(18)
where E¯and E denote downward and upward irradiances, whereas ETOA and Esurf indicate irradiances at the top of the atmosphere (TOA) and at the surface, respectively. In Tab.3, results labeled LBL1 and LBL2 correspond to previous versions of Chou (1992) and Ramaswamy & Freidenreich (1992) calculations; the differences between their respective ABS estimates were attributed by Ramaswamy & Freidenreich (1991) to the neglect of water vapor absorption in three spectral intervals (0-1000, 1000-2600, and 14500-18000 cm-1) when LBL1 results were obtained. Thus, the influence of the spectral range may reach 6 W/m2, although a fraction of this discrepancy could be associated to intrinsic differences between the line-by-line radiative codes. The influence of the line parameters compilation (around 1.7 W/m2) may be assessed by comparing the results labeled LBL1 and Chou (1992). On the other hand, the difference between results labeled Chou (1992) and Fomin et al. (1993) indicates the influence of the radiative code, around 0.7 W/m2, once the same line parameters compilation and the same spectral range were adopted. Discrepancies between ABS estimates provided by different sources would depend on Sun zenith angle as well as on atmospheric water vapor content. A more comprehensive discussion about these influences on benchmark results could be possible only after a comparison among estimates obtained under other conditions. Anyway, the influence of the spectral range seems to be more important than those associated to the line parameters compilation or to the line-by-line radiative code.
Tabs. 4 and 5 compare benchmark results compiled by Fouquart et al. (1991) with respective figures resulting from the application of selected schemes for obtaining water vapor broadband absorptances. Downward and upward shortwave irradiances were obtained by running modified versions of the radiative code developed by Davies (1982), every one corresponding to a selected scheme. In these versions, ozone absorption and molecular scattering were discarded in order to provide results consistent with the ICRCCM shortwave cases 1 to 6, whereas direct beam reflection at the ground level was retained as in the original version, that is, following a non-Lambertian behavior (see Chagas & Tarasova, 1996, for a detailed description). The solar constant Eo was assumed equal to 1367 W/m2, a very close value to those provided by recent spacecraft observations (e.g.: Mecherikunnel, 1996). In both tables, discrepancies between results labeled LBL1 and LBL2 are consistently positive, being associated mainly to the differences existing in the spectral range taken into account for water vapor absorption (Ramaswamy & Freidenreich, 1991). Results labeled LBL2 are adopted as reference in these tables because they correspond to a larger spectral range (see Tab.3).
Tab. 4 displays downward shortwave irradiance at the ground values which depend on Sun position and on water vapor absorption only. Absolute deviations due to the scheme of Ramaswamy & Freidenreich (1992) with respect to the LBL2 results are very low because this one was developed from calculations performed essentially with the same line-by-line radiative code which provided LBL2 results. Absolute deviations due to the schemes of Briegleb (1992) and of Chou & Lee (1996) have very similar magnitude (discrepancies below 1.5 W/m2), despite of a number of differences existing between these schemes. Such differences involve not only the spectral range covered and the own line-by-line radiative code employed, but also the scaling approach adopted. Absolute deviations due to the schemes of Lacis & Hansen (1974) and of Davies (1982) have also similar magnitude (discrepancies below 1.0 W/m2 between them), once both schemes were developed from the set of calculations provided by Yamamoto (1962). The discrepancies between LBL2 results and those furnished by the latter schemes are around two times more important than those associated to relatively modern schemes (Briegleb, 1992; and Chou & Lee, 1996). This fact seems to indicate that atmospheric water vapor absorption estimates can be wrong if entirely based upon old absorption data. According to the results shown in Tab.4, such systematic deviations would increase with the water vapor content and would decrease with the Sun zenith angle. Moreover, these deviations are beyond the accuracy level required for flux calculations in the scope of some climate programs, ± 10 W/m2 (Ellingson & Fouquart, 1991).
The relative accuracy of selected schemes for obtaining water vapor broadband absorptances was discussed above from the analysis of radiative quantities respective to the entire atmosphere, namely the downward irradiance at the bottom of the column and the total absorbed irradiance in the column. The vertical distribution of such absorption may be presented in terms of radiative heating rates, defined as (Liou, 1992, p.108):

(19)
where dT(p)/dt would be the time variation of the air temperature at the pressure level p if only radiative processes were present, cp indicates the specific heat at constant pressure and EN(p) denotes the net shortwave irradiance.
Fig. 1 compares heating rates for the ICRCCM shortwave cases 3 and 4, resulting from downward and upward shortwave irradiances provided by modified versions of the radiative code developed by Davies (1982), every one corresponding to a selected scheme for obtaining water vapor broadband absorptances. Profiles obtained by applying the schemes proposed by Ramaswamy & Freidenreich (1992), by Briegleb (1992) and by Chou & Lee (1996) agree relatively well (deviations of around or less than 0.1 K/day) with the respective line-by-line calculations performed by those authors. Heating rates decrease as the Sun moves away from the zenith mainly because the downward shortwave irradiance available to the atmospheric absorption reduces by a factor equal to the cosine of the Sun zenith angle.
Fig. 1 shows that the scheme proposed by Briegleb seems to be the unique one which enables realistic, unvanishing heating rates above 50 hPa when compared against benchmark results (see Fig.3 of Ramaswamy & Freidenreich (1992), Fig.4 of Briegleb (1992) or yet Fig.3 of Chou & Lee (1996)). This fact is associated to the scaling approach adopted. The modified pressure-weighted path proposed by Briegleb (1992) (see Eq.(10)) was conceived specifically for retrieving the basic features exhibited by benchmark results in the stratosphere; in contrast, scaling functions like those given by Eqs.(9) and (11) vanish when the pressure vanishes. The scheme proposed by Ramaswamy & Freidenreich (1992) provides radiative heatings which in the lower troposphere are somewhat greater than those obtained by applying the schemes of Briegleb (1992) and of Chou & Lee (1996), because different spectral ranges for water vapor absorption were assumed in the development of these schemes from line-by-line calculations (see Tab.1). The schemes proposed by Lacis & Hansen (1974) and by Davies (1982) allow very similar heating rate profiles above 300 hPa, although exhibiting some bias when compared with respective figures provided by the three other schemes. The agreement between the results labeled Lacis & Hansen (1974) and Davies (1982) above 300 hPa can be explained by the common origin of both schemes (Yamamoto, 1962). Below 300 hPa, results labeled Davies show an oscillating feature similar to that reported by Chou & Lee (1996), associated to a low value for N in Eq.(5).
The scheme proposed by Chou & Lee (1996) is hereafter adopted as reference for assessing the relative accuracy associated to the other schemes here compared. The choice of this scheme is motivated by its development from a recent compilation of absorption line parameters (HITRAN 1992 ¾ Rothman et al., 1992), together with the adoption of a scaling approach which present some conceptual advantages with respect to other schemes (Chou & Arking, 1981). Such a choice is somewhat arbitrary yet either the scheme of Briegleb (1992) or that of Ramaswamy & Freidenreich (1992) could in principle be chosen, the first by its reliability throughout the stratosphere, the latter by its wider spectral range for water vapor absorption.
Fig. 2 displays absolute deviations in heating rates with respect to the results provided by the scheme of Chou & Lee (1996), corresponding to the four other schemes compared here. ICRCCM shortwave cases 1, 3 and 5 correspond to a Sun zenith angle of 30°, whereas the cases 2, 4 and 6 correspond to an angle of 75°. In a similar way to the behavior exhibited by the own heating rates (see Fig.1), absolute deviations decrease for an increase in Sun zenith angle.
Absolute deviations in heating rates with respect to the results provided by the scheme of Chou & Lee (1996), for ICRCCM cases 1 to 6 (see Tab.2), due to the other schemes for obtaining water vapor broadband absorptances. Labels LH, D, RF, and B are the same ones employed in Fig.1 .
Figura 2 - Desvios absolutos, em taxas de aquecimento, medidos a partir dos resultados obtidos aplicando-se o esquema de Chou & Lee (1996) e referentes a outros esquemas para avaliação da absortância solar devida ao vapor d'água, para os casos 1 a 6 do programa ICRCCM (ver Tab.2). Siglas LH, D, RF e B empregadas como na Fig.1 .
Absolute deviations associated to the scheme proposed by Ramaswamy & Freidenreich (1992) can reach +0.15 K/day in the lower troposphere under relatively moist conditions (case 3), being such a bias could be at least partially explained by the spectral ranges considered for the development of this scheme and that of Chou & Lee (1996) (see Tab.1). Absolute deviations associated to the scheme proposed by Briegleb (1992) can reach -0.2 K/day in upper troposphere (case 1) and +0.1 K/day in stratosphere (cases 1, 3, and 5), mainly due to the differences between the scaling approaches adopted together with this scheme and that of Chou & Lee (1996). Absolute deviations associated to the schemes proposed by Lacis & Hansen (1974) and by Davies (1982) are negative above 300 hPa, reaching -0.5 K/day under relatively moist conditions (cases 1 and 3). Below that level, both schemes (and mainly that proposed by Davies) allow absolute deviations in heating rates which exhibit an oscillating feature around a negative value falling between -0.05 and -0.1 K/day. This feature is artificially produced by the subtraction between two heating rate profiles, one of them presenting its own oscillating feature. Sensitivity tests have shown that the amplitude of the oscillations superimposed on heating rate profiles decreases when the value of N employed in Eq.(5) is increased (Chou & Lee, 1996). Absolute deviations associated to the schemes proposed by Ramaswamy & Freidenreich (1992) and by Briegleb (1992) present also vertical profiles somewhat oscillating, but they are not so well-defined.
SUMMARY AND CONCLUSIONS
In order to infer the accuracy of water vapor broadband absorptances estimates as made at present in Brazilian centers of research and numerical weather forecasting, the schemes proposed by Lacis & Hansen (1974) and by Davies (1982) were submitted to a series of comparisons performed under standard conditions, more specifically the first six shortwave cases of the program ICRCCM (Ellingson & Fouquart, 1991). Their structure and common origin were discussed, enabling a more complete analysis of their limitations. Such a discussion as well as our results can in principle be of interest to everyone involved with climatic projections based upon general circulation models which employ these same schemes. That is the case of models running at the Bureau of Meteorology Research Centre (Melbourne, Australia), at the Geophysical Fluid Dynamics Laboratory (Princeton, New Jersey, USA), at the Goddard Institute for Space Sciences (New York, USA), at the Japan Meteorological Agency (Tokyo), and at the National Meteorological Center (Washington, USA), among other institutions (Gates, 1992).
The reliability of the scheme proposed by Lacis & Hansen (1974) can be considered low, as shown in this study from different comparisons with benchmark results (line-by-line calculations) as well as with respective evaluations provided by relatively modern schemes (Ramaswamy & Freidenreich, 1992; Briegleb, 1992; and Chou & Lee, 1996). Such low reliability has been related by several authors (Stephens, 1984; Kratz & Cess, 1985; Chou, 1986; Ramaswamy & Freidenreich, 1992) to the limits of applicability of the fit proposed by Lacis & Hansen (1974), or to the unsuitability of the extrapolation criteria adopted by Yamamoto (1962) to estimate absorptances beyond experimental conditions. In our opinion, the low reliability of that scheme can at least partially be associated to the unsuitability of the contributions resulting from Fowle (1915) for the computation of radiative heating rates (Roach, 1961), and to the questionable reliability of the measurements performed by Howard et al. (Yamanouchi & Tanaka, 1985). The same remarks apply also to the scheme proposed by Davies (1982), being that this one can provide radiative heating rate profiles associated to an oscillating, non-physical feature throughout the troposphere. Under relatively moist conditions (atmosphere models: Tropical and Mid-Latitude Summer), the deviations associated to both schemes affecting downward irradiances at the surface and total atmospheric absorption values can assume figures beyond the accuracy level required for flux calculations in the scope of some climate programs, ± 10 W/m2 (Ellingson & Fouquart, 1991). Further, radiative heating rates obtained through these schemes are significatively underestimated in the upper troposphere and throughout the stratosphere with respect to more reliable estimates.
The schemes proposed by Lacis & Hansen (1974) and by Davies (1982) enable underestimates for the water vapor absorption of solar radiation in the atmosphere, and overestimates for the available shortwave radiation at the surface. The energy budget at neighbouring levels may be affected, allowing at least a certain impact on boundary-layer processes as well as on thermodynamic stability features of the lower troposphere. The overall influence on several meteorological fields is discussed in the companion article (Souza et al., 1998, this issue).
Submetido em: 25/07/97
Revisado pelo(s) autor(es) em: 20/04/98
Aceito em: 25/04/98
ABSORÇÃO DE RADIAÇÃO SOLAR POR VAPOR D'ÁGUA NA ATMOSFERA. PARTE I: UMA COMPARAÇÃO ENTRE PARAMETRIZAÇÕES E RESULTADOS DE REFERÊNCIA
Este artigo analisa diversos aspectos relacionados à avaliação dos efeitos da absorção de radiação solar pelo vapor d'água na atmosfera, particularmente quando efetuada através de esquemas (parame-trizações) que sejam acurados e com baixo custo computacional. Estes últimos dois aspectos são pertinentes no contexto do emprego de modelos atmosféricos para a obtenção de previsões do tempo e de projeções climáticas. Atenção especial é dedicada ao esquema proposto por Lacis & Hansen (1974), dado que ele encontra-se incluído em códigos radiativos de modelos atmosféricos empregados em diferentes instituições brasileiras. A análise das diversas etapas do desenvolvimento daquele esquema permite a identificação de aspectos que têm sido até aqui menosprezados na literatura quanto à origem de sua limitada confiabilidade. No intuito de se quantificar erros sistemáticos, comparações são efetuadas frente a resultados disponíveis na literatura e obtidos empregando-se códigos radiativos capazes de levar em conta o impacto isolado de cada linha de absorção, daí sua denominação "códigos linha-a-linha". Estas comparações consideram apenas condições atmosféricas hipotéticas estabelecidas no âmbito do programa ICRCCM (Intercomparison of Radiation Codes used in Climate Models), e incluem esquemas de desenvolvimento mais recente - Briegleb (1992), Ramaswamy & Freidenreich (1992) e Chou & Lee (1996). A dispersão entre resultados obtidos com estes três esquemas tem a mesma ordem de grandeza que a diferença em relação a resultados fornecidos por códigos "linha-a-linha". O mesmo já não ocorre com os esquemas propostos por Lacis & Hansen (1974) e por Davies (1982), incluídos respectivamente no modelo atmosférico regional RAMS (Pielke et al., 1992), e no modelo de circulação geral CPTEC/COLA; estes dois esquemas proporcionam menos absorção atmosférica, e portanto maiores valores para a irradiância solar incidente à superfície do solo, quando comparados aos resultados fornecidos por códigos "linha-a-linha". A ordem de grandeza de tais erros sistemáticos (em alguns casos em torno de 20 W/m2) motiva estudos de sensibilidade como aqueles apresentados na Parte II.
- ARMBRUSTER, W. & FISCHER, J. - 1996 - Improved method of exponential sum fitting of transmissions to describe the absorption of atmospheric gases. Applied Optics, 35(12): 1931-1941.
- BLACK, T.L. - 1994 - The new NMC Mesoscale Eta Model: description and forecast examples. Weather and Forecasting, 9(2): 265-278.
- BRIEGLEB, B.P. - 1992 - Delta-Eddington approximation for solar radiation in the NCAR Community Climate Model. Journal of Geophysical Research, 97(D7): 7603-7612.
- CHAGAS, J.C.S. & TARASOVA, T.A. - 1996 - Parameterization of atmospheric radiation in the CPTEC/COLA atmospheric general circulation model. Publ. INPE-5980-NTC/327, 142pp., Instituto Nacional de Pesquisas Espaciais, Săo José dos Campos (SP).
- CHEN, C. & COTTON, W.R. - 1983 - A one-dimensional simulation of the strato-cumulus-capped mixed layer. Boundary Layer Meteorology, 25: 289-321.
- CHOU, M.-D. - 1986 - Atmospheric solar heating rate in the water vapor bands. Journal of Climate and Applied Meteorology, 25: 1532-1542.
- CHOU, M.-D. - 1992 - A solar radiation model for use in climate studies. Journal of the Atmospheric Sciences, 49(9): 762-772.
- CHOU, M.-D. & ARKING, A. - 1981 - An efficient method for computing the absorption of solar radiation by water vapor. Journal of the Atmospheric Sciences, 38: 798-807.
- CHOU, M.-D. & LEE, K.-T. - 1996 - Parameterizations for the absorption of solar radiation by water vapor and ozone. Journal of the Atmospheric Sciences, 53(8): 1203-1208.
- DAVIES, R. - 1982 - Documentation of the solar radiation parameterization in the GLAS climate model. NASA Tech. Memo. 83961, 57pp., Goddard Space Flight Center, Greenbelt, Md.
- ELLINGSON, R.G. & FOUQUART, Y. - 1991 - The Intercomparison of Radiation Codes used in Climate Models: an overview. Journal of Geophysical Research, 96(D5): 8925-8927.
- ELLINGSON, R.G., ELLIS, J. & FELS, S. - 1991 - The Intercomparison of Radiation Codes used in Climate Models: long wave results. Journal of Geophysical Research, 96(D5): 8929-8953.
- FOMIN, B.A., ROMANOV, S.V. & TROTSENKO, A.N. - 1993 - Benchmark calculations of solar radiation transfer characteristics in a clean, clear atmosphere by the method of direct integration. Izv. RAN, Atmos. Oceanic Phys., 29(1): 50-59.
- FOUQUART, Y., BONNEL, B. & RAMASWAMY, V. - 1991 - Intercomparing shortwave radiation codes for climate studies. Journal of Geophysical Research, 96(D5): 8955-8968.
- FOWLE, F.E. - 1915 - The transparency of aqueous vapor. Astrophysical Journal, 42: 394-411.
- GATES, L.W. - 1992 - AMIP: the Atmospheric Model Intercomparison Project. Bulletin of the American Meteorological Society, 73(12): 1962-1970.
- HARDIKER, V. - 1997 - A global numerical weather prediction model with variable resolution. Monthly Weather Review, 125: 59-73.
- HOWARD, J.N., BURCH, D.E. & D. WILLIAMS - 1956a - Infrared transmission of synthetic atmospheres. I. Instrumentation. Journal of the Optical Society of America, 46(3): 186-190.
- HOWARD, J.N., BURCH, D.E. & WILLIAMS, D. - 1956b - Infrared transmission of synthetic atmospheres. III. Absorption by water vapor. Journal of the Optical Society of America, 46(4): 242-245.
- KRATZ, D.P., & CESS, R.D. - 1985 - Solar absorption by atmospheric water vapor: a comparison of radiation models. Tellus, 37B: 53-63.
- LACIS, A.A., & HANSEN, J.E. - 1974 - A parameterization for the absorption of solar radiation in the Earth's atmosphere. Journal of the Atmospheric Sciences, 31: 118-133.
- LIOU, K.-N. - 1992 - Radiation and Cloud Processes in the Atmosphere: Theory, Observation, and Modelling. Oxford University Press, 487 pp.
- McDONALD, J.E. - 1960 - Direct absorption of solar radiation by atmospheric water vapor. Journal of Meteorology, 17: 319-328.
- MECHERIKUNNEL, A.T. - 1996 - Solar total irradiance observations from spacecraft: 1992-1993. Journal of Geophysical Research, 101(A8): 17073-17079.
- PIELKE, R.A., COTTON, W.R., WALKO, R.L., TREMBACK, C.J., LYONS, W.A., GRASSO, L.D., NICHOLLS, M.E., MORAN, M.D., WESLEY, D.A., LEE, T.J. & COPELAND, J.H. - 1992 - A comprehensive meteorological modeling system - RAMS. Meteorology and Atmospheric Physics, 49(1-4): 69-91.
- RAMASWAMY, V. & FREIDENREICH, S.M. - 1991 - Solar radiative line-by-line determination of water vapor absorption and water-cloud extinction in inhomogeneous atmospheres. Journal of Geophysical Research, 96(D5): 9133-9157.
- RAMASWAMY, V. & FREIDENREICH, S.M. - 1992 - A study of broadband parameterizations of the solar radiative interactions with water vapor and water drops. Journal of Geophysical Research, 97(D11): 11487-11512.
- ROACH, W.T. - 1961 - The absorption of solar radiation by water vapour and carbon dioxide in a cloudless atmosphere. Quarterly Journal of the Royal Meteorological Society, 87: 364-373.
- ROTHMAN, L.S., GAMACHE, R.R., BARBE, A., GOLDMAN, A., GILLIS, J.R., BROWN, L.R., TOTH, R.A., FLAUD, J.-M. & CAMY-PEYRET, C. - 1983 - AFGL atmospheric absorption line parameters compilation: 1982 edition. Applied Optics, 22: 2247-2256.
- ROTHMAN, L.S., GAMACHE, R.R., GOLDMAN, A., BROWN, L.R., TOTH, R.A., PICKETT, H.M., POYNTER, R.L., FLAUD, J.-M., CAMY-PEYRET, C., BARBE, A., HUSSON, N., RINSLAND, C.P. & SMITH, M.A.H. - 1987 - The HITRAN database: 1986 edition. Applied Optics, 26(19): 4058-4097.
- ROTHMAN, L.S., GAMACHE, R.R., TIPPING, R.H., RINSLAND, C.P., SMITH, M.A.H., BENNER, D.C., DEVI, V.M., FLAUD, J.-M., CAMY-PEYRET, C., PERRIN, A., GOLDMAN, A., MASSIE, S.T., BROWN, L.R. & TOTH, R.A. - 1992 - The HITRAN molecular database: editions of 1991 and 1992. Journal of Quantitative Spectroscopy and Radiative Transfer, 48(5/6): 469-507.
- SATO, N., SELLERS, P.J., RANDALL, D.A., SCHNEIDER, E.K., SHUKLA, J., KINTER III, J.L., HOU, Y.-T. & ALBERTAZZI, E. - 1989 - Effects of implementing the Simple Biosphere Model in a general circulation model. Journal of the Atmospheric Sciences, 46(18): 2757-2782.
- SOUZA, E.P., SILVA DIAS, P.L., PLANA-FATTORI, A. & CHAGAS, J.C.S. - 1998 - Absorption of Solar Radiation by Water Vapor in the Atmosphere. Part II: Sensitivity Tests with a General Circulation Model. Revista Brasileira de Geofísica,15 (3): 291-305.
- STEPHENS, G.L. - 1984 - The parameterization of radiation for numerical weather prediction and climate models. Monthly Weather Review, 112: 826-867.
- WANG, W.-C. - 1976 - A parameterization for the absorption of solar radiation by water vapor in the earth's atmosphere. Journal of Applied Meteorology, 15: 21-27.
- YAMAMOTO, G. - 1962 - Direct absorption of solar radiation by atmospheric water vapor, carbon dioxide and molecular oxygen. Journal of the Atmospheric Sciences, 19: 182-188.
- YAMANOUCHI, T. & TANAKA, M. - 1985 - Absorption properties of the near-infrared water vapor bands. Journal of Quantitative Spectroscopy and Radiative Transfer, 34(6): 463-472.
Publication Dates
-
Publication in this collection
08 June 2001 -
Date of issue
Nov 1997
History
-
Accepted
25 Apr 1998 -
Reviewed
20 Apr 1998 -
Received
25 July 1997