Abstract
Abstract - An Aerated Submerged Biological Filter was used to promote biological nitrification of a synthetic saline wastewater. Black PVC corrugated plates were used to make the structured packing of the 6 liter reactor. Nitrate, nitrite, and ammonia concentrations, pH and DO were periodically measured, according to APHA (1985). In spite of the deleterious effect of salinity, it was possible to obtain nitrogen removal efficiencies as high as 95% for a 25 g/L salt concentration after a three-day reaction in the batch reactor. Continuous operation using a NaCl concentration of 25 g/L was tested using three different hydraulic retention times: 7, 15, and 25 hours. An increase in ammonia removal was observed when the retention time was increased from 7 to 15 hours. However, no further notable increase was obtained for a 25 hour retention time, showing that nitrogen removal tends towards a maximum limit of about 80%.
Nitrification; submerged bed; saline wastewater
NITRIFICATION OF SALINE EFFLUENTS
M.F. Rosa 1 , R.T. Albuquerque 2 , J.M.O. Fernandes 2 , S.G.F. Leite 2 and R.A. Medronho 2
1 CTAA/EMBRAPA - Av. das Américas, 29501, Pedra de Guaratiba - 23020-470 - Rio de Janeiro/RJ - Brazil (Corresponding Address) - Phone number: 55 (021) 410-1353 - Fax Number: 55 (021) 410-1090
2 Escola de Química/Universidade Federal do Rio de Janeiro - Rio de Janeiro/RJ - Brazil
(Received: October 8, 1996; Accepted: April 8, 1997)
ABSTRACT - An Aerated Submerged Biological Filter was used to promote biological nitrification of a synthetic saline wastewater. Black PVC corrugated plates were used to make the structured packing of the 6 liter reactor. Nitrate, nitrite, and ammonia concentrations, pH and DO were periodically measured, according to APHA (1985). In spite of the deleterious effect of salinity, it was possible to obtain nitrogen removal efficiencies as high as 95% for a 25 g/L salt concentration after a three-day reaction in the batch reactor. Continuous operation using a NaCl concentration of 25 g/L was tested using three different hydraulic retention times: 7, 15, and 25 hours. An increase in ammonia removal was observed when the retention time was increased from 7 to 15 hours. However, no further notable increase was obtained for a 25 hour retention time, showing that nitrogen removal tends towards a maximum limit of about 80%.
KEYWORDS: Nitrification, submerged bed, saline wastewater.
INTRODUCTION
Effluents containing nitrogenous compounds must be treated in order to reduce the release of ammonium-N into rivers and lakes, because this causes oxygen demand and bad odour, is toxic to aquatic life, increases the amount of chlorine necessary for the disinfection of the public water supply and leads to eutrophication, modifying the biota and creating an imbalance in the aquatic life chain.
With increasing requirements for effluent quality, it is becoming necessary to incorporate a process for nitrogen control. Nitrogenous compounds can be removed by ion exchange, dialysis, stripping and reverse osmosis. However, these are expensive processes. Biological nitrification and denitrification has been reported as an economical and efficient treatment alternative for water treatment.(Soares et al., 1988; Prakasam and Loehr, 1972).
Biological nitrification is performed by two bacterial populations: first, the Nitrosomonas group oxidizes ammonium-N to nitrite-N, which is subsequently converted to nitrate-N by the Nitrobacter group (Painter, 1970).
Hypersaline wastes are generated during industrial activities that include chemical manufacturing and oil and gas production. These wastes are difficult to treat using conventional biological treatments, since the salinity reduces the flocculation ability and, consequently, the sludge decantability, affecting the Biochemical Oxygen Demand (BOD) removal efficiency and significantly hindering the nitrification process (Lawton and Eggert., 1957; Dalmacija et al., 1996). Both the extent to which salinity affects the treatment and the minimum quantity which causes a reduction in process efficiencies are not yet totally understood.
The present study evaluates the biological nitrification in aerated reactors for removal of ammonium-N from a synthetic saline effluent.
MATERIALS AND METHODS
Flora Activation and Acclimation
The mixed flora used was sampled at the nitrification step at the wastewater treatment plant in a Brazilian oil refinery (REPAR/ PETROBRAS). The sludge presented a total suspended solid concentration of 12180 mg/L and a fixed suspended solid concentration of 2120 mg/L. It first passed first through an activation step and then was acclimatized to the saline effluent.
Erlenmeyer flasks containing 100 mL of specific culture medium for Nitrosomonas and Nitrobacter populations were inocculated with 10mL of sludge and maintained in a shaker at a constant temperature (30oC). Analogous experiments without sludge were carried out as a blank test. Transferences were done weekly, in order to enrich the microflora. The values for ammonium-N, nitrite-N and nitrate-N were periodically measured in order to evaluate the performance of the microflora. The culture medium contained Na2HPO4 (13.5 g/L), KH2PO4 (0.7 g/L), MgSO4.7H2O (0.1 g/L), NaHCO3 (0.5 g/L), FeCl3.6H2O (0.014 g/L) and CaCl2.2H2O (0.18 g/L). To activate the Nitrobacter population, 500 mg/L NaNO2 were added to the medium and to activate the Nitrosomonas population, 500 mg/L NH4Cl were used (American Society for Microbiology, 1981). After several subsequent transferences bacteria were allowed to grow in Petri dishes, using the same medium with 5% w/v agar.
In order to acclimatize bacteria to the saline effluent several studies on aerated bench scale reactors were done employing suspended biomass. The synthetic effluent containing growing NaCl concentrations was added to the activated biomass. At the end of each ten-day cycle, aeration was ceased, the sludge was sedimented and the effluent was replaced with one with a higher NaCl concentration.
Effluent Characterization
The composition of the synthetic effluent employed is: MgSO4.7H2O (140mg/L), CaCl2.2H2O (10mg/L), NaCl.2H2O (300mg/L), KH2PO4 (50mg/L), FeSO4 (7,5mg/L), NH4Cl (157,3mg/L), NaHCO3 (500mg/L), Na2CO3 (1000mg/L), MnSO4.H2O (0,0675mg/L), CuSO4 (0,00425mg/L), Na2MoO4.2H2O (0,00242mg/L), ZnSO4.7H2O (0,07mg/L) (Nesaratnam, 1985).
Batch Operation
All experiments were conducted in a bench scale apparatus. Initially two different systems were tested: an Aerated Submerged Biological Filter (ASBF) with fixed biomass and a simple aerated reactor with suspended biomass. In both cases the biomass inoculated had been previously acclimatized. Aeration was done by directly injecting air through a porous medium at the bottom of the reactors, always maintaining the dissolved oxygen level near saturation. NaCl concentrations tested were in the range of 0 to 50 g/L.
Continuous Operation
Only the ASBF was tested at this stage, because it had proved to be more efficient in the previous tests. The system employed consisted of two packed reactors in series with ascending flow (Figure 1). Each reactor was a retangular box in acrylic with a pyramidal base and a volume of 6 L. In this type of reactor, the biofilm support remains submerged and air is introduced via diffusers. The filter is a three-phase system where the adsorption and diffusion phenomena are expected to occur in the biofilm attained to the packing material. This system combines the mechanisms of fixed and suspended biomass, with the predominance of removal due to the biofilm. The reactor more closely resembles the complete mixture type, because of the introduction of air that promotes a good homogenization of the liquid.
Figure 1: Diagram of the experimental equipment.
The support material for biofilm development chosen was a comercial product (Biodek 60) consisting of blocks of cross flow corrugated plates of black PVC with a high specific area (42 ft2/ft3). Its good flux distribution and oxygen transfer ability lead to high removal efficiencies. Additionally it has high porosity (>95%), produces a small pressure drop and allows operation with in a wide range of hydraulic loads. It can be easily installed and its operation, control and washing are quite simple.
During continuous operation saline effluent containing 25 g/L NaCl was fed to the reactor and different hydraulic retention times were tested.
Analytical Procedures
The majority of the analyses done for evaluating system performance was based on the procedures recommended by APHA (1985). The ammonium and nitrite levels were measured directly with ion-selective electrodes from Orion and Ingold, respectively. The nitrate levels were measured with ion-selective electrodes from Ingold and reference electrode from Analion. The pH was measured with a potentiometer (Analion, model PM600) and the dissolved oxygen was monitored with a membrane electrode (Digimed, model DMO-2). Temperature was maintained at approximately 28oC. Samples were withdrawn periodically and the microflora was allowed to grow in solid medium in Petri dishes, as described in 2.1. An optical microscope (Karl Zeiss, model STEMI - SV11) with camera was used to observe the microbial population.
RESULTS AND DISCUSSION
Microflora Activation
Ammonium, nitrite and nitrate values measured during the activation stage are shown in Figures 2 and 3.
Figure 2: Microflora activation - Nitrosomonas
Figure 3: Microflora activation - Nitrobacter
In Figure 2 it can be noticed that the ammonium-N was converted to nitrite-N in the flasks containing biological sludge, giving evidence of the action of the Nitrosomonas population. Figure 3 also shows the good performance of the microflora in converting nitrite to nitrate.
Considering that in biological systems the ammonium-N can also be used for microbial growth, that ammonium stripping may occur and that N2O can be produced, the nitrite and nitrate values demonstrate that nitrification corresponded to 80% of the global process. The tests without sludge confirm that this conversion has been essentially due to the microflora present in the sludge.
At the end of this stage different reddish brown colonies were detected in the Petri dishes where the microbial population was allowed to grow. Microscopic observations showed the existence of a typical microflora. Figures 4 and 5 are potographs of the colonies and of a typical microscopic preparation.
Batch Operation
In all experiments there was a slight reduction in the pH values within the range of 7.0 to 7.5, typical behaviour in nitrification. Dissolved oxygen was always within the range of 80% to 85% of the saturation. Figure 6 shows the ammonium removal efficiency as a function of the operating time for the two different reactors and for two saline concentrations.
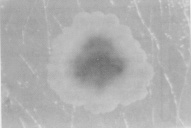
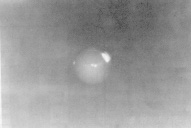
Figure 6: Effect of reactor type and salinity on ammonium removal.
Figure 6 shows that the best performance was obtained when using the fixed biomass reactor (ASBF) with a NaCl concentration of 25g/L. For instance, the time necessary to attain a 90% ammonium conversion in the fixed biomass reactor (ASBF) is just 25% of that required by the suspended biomass reactor. Based on these results, the NaCl concentration of 25g/L was chosen for the experiments with the continuous reactor.
The results in Figure 6 also show that an increase in salinity leads to a reduction in process efficiency, confirming the studies developed by Kincannon and Gaudy (1966). They noticed that when working with a Rotating Biological Contactor (RBC), removal of ammonium-N was only possible at a maximum concentration corresponding to 10% of that of seawater.
Additionally, the results show a better performance with the ASBF, confirming that the fixed film presents a high load shock resistance, and the microbial immobilization permitted operation at a high biomass concentration, resulting in better efficiencies (Bryers and Characklis,1982 and Canter and Perret,1994).
During the experiments the biofilm formation was visible, sustaining a good dissolved oxygen level in the reactor. Figure 7 shows the biofilm in an eletronic microscope (JSM 5300 Scan Mycroscope).
Macroscopically it was also possible to observe changes in the biofilm behaviour, with a reduction of the adhering capacity as the saline concentration increased.
Figure 8 shows the ammonium, nitrite and nitrate profiles for a typical experiment operating the ASBF batchwise. The decreasing ammonium concentration, the low nitrite levels and the increasing nitrate concentrations are evidence of the different phases of the nitrification process.
Continuous Operation
Because of the superior perfomance of the ASBF observed previously, only this type of reactor was investigated under continuous operation. Different residence times were tested with a NaCl concentration of 25 g/L. Generally the system behaviour was stable, rapidly attaining the steady state. Figure 9 shows the influence of different hydraulic retention times on the ammonium removal efficiency.
It can be seen that removal efficiency increases from 53% to 80% when the hydraulic retention time is changed from 7 to 15 hours. However, it increases from 80% to only 82% when the retention time increases from 15 to 25 hours which shows that there is a biomass adaptation limit. This is probably due to the fact that high salt concentrations tend to disrupt normal metabolic functions and reduce degradation kinetics.
Figure 10 represents the typical results for the ASBF, operating continuously with a NaCl concentration of 25 g/L and a 15-hour retention time.
Figure 7: Biofilm formation.
Figure 8: Nitrification of saline effluent - Bach operation (25g/L NaCl).
Figure 9: Effect of retention time on ammonium removal.
CONCLUSIONS
The results confirmed that aerated submerged biological filters promote more stability and operational flexibility. When operated continuously, the reactor can reach the steady state within the first day of operation. In this case, removal efficiencies of approximately 80% were attained with a global retention time of just 15 hours. For batchwise operation with a NaCl concentration of 25g/L, the period of time necessary to attain a 90% ammonium conversion in the fixed biomass reactor (ASBF) was just 25% of that required by the suspended biomass reactor. The microbial immobilization enabled operation at high biomass concentration and consequently promoted better efficiencies.
It was also possible to observe that an increase in salinity leads to reduced ammonium removal efficiencies. For batchwise operation of the ASBF, after three days it was possible to verify that the removal efficiency fell from 95% to 50% when the NaCl concentration increased from 25 to 50g/L.
ACKNOWLEDGEMENT
We acknowledge the support received from the FUJB (José Bonifácio University Foundation), Brazil.
REFERENCES
American Society for Microbiology, Manual of Methods for General Bacteriology (1981).
Belkin, S.; Brenner, A. and Abeliovich, A., Biological Treatment of A High Salinity Chemical Industrial Wastewater. Water Science Technology, Vol. 27, No. 7-8, pp.105-112 (1993).
Bryers, J.D. and Characklis, W.G., Process Governing Primary Biofilm Formationn. Biotechnology and Bioengineering, V.24, pp. 2451-247 (1982).
Canter, J.P. and Perret J.M., Biological Aerated Filters: Assessment Of The Process Based on 12 Sewage Treatment Plants. Wat. Sci. Tech. Vol. 29, No10-11, pp. 13-22 (1994).
Dalmacija, B.; Karlovic, E.; Tamas, Z. and Miskovic, D., Purification of High-Salinity Wastewater by Activated Sludge Process. Water Research, Vol. 30, No. 2, pp. 295-298 (1996).
Lawton, G.W. and Eggert, C.V., Effects of High Sodium Chloride Concentrations on Trickling Filter Slimes. Sewage and Industrial Wastes, 29, 11, 1228 (Nov. 1957).
Kincannon, D.F. and Gaudy Jr., A.F., Some Effects of High Salt Concentrations on Activated Sludge. Journal of Water Pollution Control Federation, 38, pp. 1148-1159 (1966).
Klemetson, S.L. and Lang, M.E., Treatment of Salin Wastewaters Using a Rotating Biological Contactor. Journal of Water Pollution Control Federation, 56, pp. 1254-1259 (1984).
Nesaratnam, S.T. and Ghobrial, F.H., Biological Treatment of Industrial and Sanitary Wastewaters. Conservation & Recyclig. Vol. 8, No 1/2, pp. 135-142, (1985).
Painter, H.A., A Review of Literature on Inorganic Nitrogen Metabolism in Microorganisms. Water Research, Vol.4, pp.393-450 (1970).
Prakasam, P.B.S. and Loehr, R.C., Microbial Nitrification and Denitrification in Concentrated Wastes. Water Research Vol. 6, pp. 859-869 (1972).
Ryhiner, G.; Birou, B. and Gros, H., The Use Of Submerged Structured Packings In Biofilm Reactors For Wastewater Treatment. Wat. Sci. Tech. Vol. 26, No. 3-4, pp. 723-731 (1992).
Soares, M.I.M.; Belkin, S. and Abeliovich, A., Biological Groundwater Denitrification: Laboratory Studies, Water Science Technolgy Vol. 20, pp. 189-195 (1988).
Standard Methods for the Examination of Water and Wastewater, 16th Edition, (APHA) American Public Health Association, Washington, D.C. (1985).
- Belkin, S.; Brenner, A. and Abeliovich, A., Biological Treatment of A High Salinity Chemical Industrial Wastewater. Water Science Technology, Vol. 27, No. 7-8, pp.105-112 (1993).
- Bryers, J.D. and Characklis, W.G., Process Governing Primary Biofilm Formationn. Biotechnology and Bioengineering, V.24, pp. 2451-247 (1982).
- Canter, J.P. and Perret J.M., Biological Aerated Filters: Assessment Of The Process Based on 12 Sewage Treatment Plants. Wat. Sci. Tech. Vol. 29, No10-11, pp. 13-22 (1994).
- Dalmacija, B.; Karlovic, E.; Tamas, Z. and Miskovic, D., Purification of High-Salinity Wastewater by Activated Sludge Process. Water Research, Vol. 30, No 2, pp. 295-298 (1996).
- Lawton, G.W. and Eggert, C.V., Effects of High Sodium Chloride Concentrations on Trickling Filter Slimes. Sewage and Industrial Wastes, 29, 11, 1228 (Nov. 1957).
- Kincannon, D.F. and Gaudy Jr., A.F., Some Effects of High Salt Concentrations on Activated Sludge. Journal of Water Pollution Control Federation, 38, pp. 1148-1159 (1966).
- Klemetson, S.L. and Lang, M.E., Treatment of Salin Wastewaters Using a Rotating Biological Contactor. Journal of Water Pollution Control Federation, 56, pp. 1254-1259 (1984).
- Nesaratnam, S.T. and Ghobrial, F.H., Biological Treatment of Industrial and Sanitary Wastewaters. Conservation & Recyclig. Vol. 8, No 1/2, pp. 135-142, (1985).
- Painter, H.A., A Review of Literature on Inorganic Nitrogen Metabolism in Microorganisms. Water Research, Vol.4, pp.393-450 (1970).
- Prakasam, P.B.S. and Loehr, R.C., Microbial Nitrification and Denitrification in Concentrated Wastes. Water Research Vol. 6, pp. 859-869 (1972).
- Ryhiner, G.; Birou, B. and Gros, H., The Use Of Submerged Structured Packings In Biofilm Reactors For Wastewater Treatment. Wat. Sci. Tech. Vol. 26, No. 3-4, pp. 723-731 (1992).
- Soares, M.I.M.; Belkin, S. and Abeliovich, A., Biological Groundwater Denitrification: Laboratory Studies, Water Science Technolgy Vol. 20, pp. 189-195 (1988).
- Standard Methods for the Examination of Water and Wastewater, 16th Edition, (APHA) American Public Health Association, Washington, D.C. (1985).
Publication Dates
-
Publication in this collection
09 Oct 1998 -
Date of issue
June 1997
History
-
Received
08 Oct 1996 -
Accepted
08 Apr 1997