Abstract
We evaluated the conditions to produce lipase in solid-state cultivation using a recently isolated strain of Aspergillus brasiliensis 157f in bioreactors of different configurations: static flat-bed, plugged-flow bed with forced water-saturated aeration, and pilot-scale rotating drum bioreactor, using malt bagasse as substrate. Lipase production was optimized applying experimental design analysis, which showed optima parameters defined as pH 7.7, addition of 11.3 % of soybean oil to the medium, and culture temperature of 32.7 oC, in static flat-bed. The highest enzyme activity (9.8 U.g-1 substrate) was obtained in the plugged-flow bed with forced water-saturated aeration. The fermented culture medium was lyophilized to create a solid enzymatic preparation (SEP), which was used to test the possibility of using this cheap biocatalyst in bioreactors to mediate esterification and transesterification reactions. SEP presented lipase activities of 7.35 U.g-1 substrate, indicating the possibility of further enhancing aspects of the use of such biocatalyst.
Key words
Aspergillus brasiliensis; brewery’s spent malt; lipase production; Solid-state cultivation; transesterification reaction
INTRODUCTION
Lipases (triacylglycerol hydrolases, EC 3.1.1.3), are enzymes that catalyze the hydrolysis of triglycerides, producing free fatty acids and glycerol. They also can catalyze alcoholysis, esterification, and transesterification of fatty acids (Treichel et al. 2010TREICHEL H, DE OLIVEIRA D, MAZUTTI MA, DI LUCCIO M & OLIVEIRA JV. 2010. A review on microbial lipases production. Food Bioprocess Technol 3: 182-196.). Based on their versatility, selectivity, moderate reaction conditions, and susceptibility to immobilization, lipases are extremely interesting from a commercial point of view. Currently, these enzymes are used in various industrial processes such as the production of foods, pharmaceuticals, cosmetics, detergents, and biofuels, among other uses (Treichel et al. 2010TREICHEL H, DE OLIVEIRA D, MAZUTTI MA, DI LUCCIO M & OLIVEIRA JV. 2010. A review on microbial lipases production. Food Bioprocess Technol 3: 182-196., Choudhury & Bhunia 2015CHOUDHURY P & BHUNIA B. 2015. Industrial Application of Lipase: A Review. Biopharm J 1: 41-47., Gupta et al. 2015GUPTA R, KUMARI A, SYAL P & SINGH Y. 2015. Molecular and functional diversity of yeast and fungal lipases: Their role in biotechnology and cellular physiology. Prog Lipid Res 57: 40-54.).
The world market for enzymes in 2014 reached 4.2 billion dollars, projected to grow at rate of 7 % per year between 2015 and 2020, and the lipase market is expected to reach 590.5 million dollars in 2020 (Chauhan et al. 2016CHAUHAN M, YENNAMALLI RM & GARLAPATI VK. 2016. Biochemical characterization and molecular modeling of a unique lipase from Staphylococcus arlettae JPBW-1. Eng Life Sci 16: 762-768.). During the last decade, lipases have been studied as a biocatalyst for the production of biodiesel, for its importance as biofuel. Some advantages of using lipases for biodiesel production are based on the use of acid oils without productivity reduction. The enzymatic route also generates pure glycerol as by-product and biodiesel of better quality compared to chemical synthesis, and the possibility of using ethanol, milder reaction conditions, and no need to neutralize the final product (De Castro et al. 2012DE CASTRO SM & DE CASTRO AM. 2012. Assessment of the Brazilian potential for the production of enzymes for biofuels from agroindustrial materials. Biomass Convers Biorefinery 2: 87-107.).
Currently, lipases are produced from microbial sources because of high productivities achieved in bioprocesses (Hasan et al. 2006HASAN F, SHAH AA & HAMEED A. 2006. Industrial applications of microbial lipases. Enzyme Microb Technol 39: 235-251.), either in submerged or solid-state cultivations. Solid-state cultivation (SSC) has some interesting characteristics such as the possibility of using agro-industrial waste or residues and the process is conducted in the absence of free water and buffering effect, thus reducing the costs of enzyme production and recovery (Edwinoliver et al. 2010EDWINOLIVER NG, THIRUNAVUKARASU K, NAIDU RB, GOWTHAMAN MK, KAMBE TN & KAMINI NR. 2010. Scale up of a novel tri-substrate fermentation for enhanced production of Aspergillus niger lipase for tallow hydrolysis. Bioresour Technol 101: 6791-6796.). These advantages are fully exploited when SSC is conducted using fungi, based on the high capacity of these microorganisms to colonize the substrate and produce lytic enzymes (Thomas et al. 2013THOMAS L, LARROCHE C & PANDEY A. 2013. Current developments in solid-state fermentation. Biochem Engineer J 81: 146-161., Ashok et al. 2017ASHOK A, DORIYA K, RAO DRM & KUMAR DS. 2017. Design of solid state bioreactor for industrial applications: An overview to conventional bioreactors. Biocatal Agric Biotechnol 9: 11-18.). The majority of fungal lipases are usually excreted to the medium, which eases the unit operations of extraction and reducing production costs (Silva et al. 2005SILVA WOB, MITIDIERI S, SCHRANK A & VAINSTEIN MH. 2005. Production and extraction of extracellular lipase from entomopathogenic fungus Metarhizium anisopliae (Clavicipitaceae: Hypocreales). Process Biochem 40: 321-326.). Aspergillus is among the most important fungal lipase producers, with great potential as source of lipase, ability to degrade plant polysaccharides and interesting properties for industrial application (Pokorny et al. 1994POKORNY D, FRIEDRICH J & CIMERMAN A. 1994. Biotechnol Lett 16: 359-362., Contesini et al. 2010CONTESINI F, LOPES D, MACEDO G & NASCIMENTO M. 2010. Aspergillus sp. lipase: Potential biocatalyst for industrial use. J Mol Catal B Enzym 67: 163-171.). This genus is found worldwide and has several known lipase producers species being generally recognized as safe (GRAS), acknowledged by the Food and Drug Administration (FDA) in USA (Contesini et al. 2010CONTESINI F, LOPES D, MACEDO G & NASCIMENTO M. 2010. Aspergillus sp. lipase: Potential biocatalyst for industrial use. J Mol Catal B Enzym 67: 163-171.). Furthermore, the product of a SSC can be directly used as a solid enzymatic preparation (SEP), which is a technique in which the cultivated solid medium can be directly used for catalytic purposes, being reported to be simple and easy to perform, showing good results, having a low cost of production, and maintaining enzymatic activity over reuses (Rosa et al. 2006ROSA DR, CAMMAROTA MC, MARIA D & FREIRE G. 2006. Preparation Produced by Penicillium restrictum in Activated Oil and Grease 23: 814-823., Salum et al. 2010SALUM TFC, VILLENEUVE P, BAREA B, YAMAMOTO CI, CÔCCO LC, MITCHELL DA & KRIEGER N. 2010. Synthesis of biodiesel in column fixed-bed bioreactor using the fermented solid produced by Burkholderia cepacia LTEB11. Process Biochem 45: 1348-1354.).
Malt bagasse (MB), also known as spent brewery’s malt, is the largest by-product of the brewing industry, representing around 85 % of its waste generation (Mussatto et al. 2006MUSSATTO SI, DRAGONE G & ROBERTO IC. 2006. Brewers’ spent grain: Generation, characteristics and potential applications. J Cereal Sci 43: 1-14.). Approximately 20 kg of spent malt is generated for each hectoliter of beer produced (Mussatto et al. 2006MUSSATTO SI, DRAGONE G & ROBERTO IC. 2006. Brewers’ spent grain: Generation, characteristics and potential applications. J Cereal Sci 43: 1-14., Thiago et al. 2014THIAGO R, PEDRO PM DE M & ELIANA FCS. 2014. Solid wastes in brewing process: A review. J Brew Distill 5: 1-9.). Currently, is estimated an annual production of two billion hectoliters of beer worldwide, with China being the largest producer, followed by USA and Brazil (Kirin 2017KIRIN. 2017. Kirin Beer University Report Global Beer Production by Country in 2016. [accessed 2018 Aug 15]. http://www.kirinholdings.co.jp/english/news/2017/0810_01.html.
http://www.kirinholdings.co.jp/english/n...
), representing a potential of forty million tons of spent malt available that could be used in biorefinery processes every year, at low or no cost (Aliyu & Bala 2013ALIYU S & BALA M. 2013. Brewer’s spent grain: A review of its potentials and applications. African J Biotechnol 10: 324-331.).
Considering these aspects, the objective of this study was to evaluate optimal conditions for lipase production using a new strain of Aspergillus brasiliensis 157f, isolated by our group, growing under solid-state cultivation using spent malt bagasse. A central composite design analysis was performed to obtain optimal conditions of cultivation in bench scale bioreactors (static flat-bed). The optimal conditions were used for cultivation test in bioreactors with forced aeration (plugged-flow bed with forced water-saturated aeration), and in a pilot-scale rotating drum bioreactor using intermittent agitation. Finally, a solid enzymatic preparation was tested for its lipase activity as a preliminary study of possible applications of this cheap enzymatic preparation in reaction requiring the action of lipases.
MATERIALs AND METHODS
Microorganism, spent malt bagasse, and chemicals
Aspergillus brasiliensis 157f was isolated by our group from soil samples in Brazil and was used for the solid-state cultivations because it demonstrated the ability to produce lipase (Da Silva Menezes et al. 2017DA SILVA MENEZES B, ROSSI DM & AYUB MAZ. 2017. Screening of filamentous fungi to produce xylanase and xylooligosaccharides in submerged and solid-state cultivations on rice husk, soybean hull, and spent malt as substrates. World J Microbiol Biotechnol 33: 58-72.). This strain was isolated, genetically identified, and physiologically characterized for the production of several enzymes in a previous work, and certified lyophilized stocks are kept in our laboratory (Bioteclab, UFRGS, Porto Alegre, Brazil) (Da Silva Menezes et al. 2017DA SILVA MENEZES B, ROSSI DM & AYUB MAZ. 2017. Screening of filamentous fungi to produce xylanase and xylooligosaccharides in submerged and solid-state cultivations on rice husk, soybean hull, and spent malt as substrates. World J Microbiol Biotechnol 33: 58-72.). For maintenance, the fungus was grown on Petri dishes containing potato dextrose (34 g.L-1) and agar (15 g.L-1) medium for 7 days at 37 oC for spore collection. The substrate used was spent malt bagasse (kindly provided by Ralf Beer Brewery Company, Alvorada, Brazil) collected immediately after the mashing process. Spent malt was stocked at -20 oC until utilization, without any further processing. The substrate was characterized for its content of lipids, reducing sugars, ashes, humidity, total proteins, cellulose, hemicelluloses, and lignin. Soybean oil, which was added to the substrate for cultivations as inducer of lipase production, was the commercial refined cooking oil bought on a local market. All chemicals used in this research were of analytical grade and purchased from Sigma-Aldrich (São Paulo, Brazil) unless otherwise informed.
Solid-state cultivation
Solid-state cultivation (SSC) for the experimental design was performed using 125 mL Erlenmeyer flasks containing 10 g of substrate (malt bagasse and oil), in which the percentages of each substrate were determined by experimental design (see below). Flasks containing substrate were autoclaved at 121 oC for 15 min, then, corresponding autoclaved buffer and autoclaved soybean oil were added. Buffers were added to provide maximum amount of water to be absorbed by the malt bagasse but avoiding any free water to accumulate, which was found to be 30 % (mass fraction to malt bagasse). Inoculum was prepared by pouring 10 mL of Tween 80 (0.01 % mass fraction) to a plate previously colonized by the fungus (as prepared in 1.1.), and smoothly scraping for spore collection. The resulted spore suspension was centrifuged (4,500 g, 20 oC, 5 min), and resuspended in sterile distilled water. Spores were counted in Neubauer chamber and inoculum was made by addition of 1•106 spores per gram of malt bagasse.
Experimental design and method validation
Central composite design (CCD) was employed as experimental design to evaluate the percentage of soybean oil, the cultivation temperature, and the pH of buffer used at the start of cultivation. Two different buffers were used: phosphate buffer 0.2 M for pH 6.0 and higher, and acetate buffer 0.2 M for pH lower than 6.0. Extracellular lipase activity was measured at different times of cultivation (0, 24, 48, 72, and 96 h), and the time of sampling with highest activity was used as response in the CCD analysis. Independent variables and their level are shown in Table I. This experimental design consisted of 16 treatments, with 8 factorial points, 6 axial points, and 2 central points. Experimental design and analysis were evaluated using STATISTICA software 10.0 (Statisoft, USA).
Variables analyzed in the CCD showing the levels and the real values for the experimental design to optimize lipase production by A. brasiliensis 157f cultivated in malt bagasse.
Lipase essay
For lipase extraction, 5 g of cultivated substrate was added to 25 mL of phosphate buffer (pH 7, 0.1 M), incubated at 37 ºC, 180 rpm, 30 min and filtered through qualitative filter paper (80 g), resulting in a crude enzymatic extract. Lipase assay was performed according to the literature (Burkert et al. 2004), with some modifications as follows. 2.5 mL of olive oil emulsion (25 % volume fraction of olive oil dispersed in a solution of Arabic gum 70 g.L-1) was added to 1 mL of acetate buffer (pH 5, 0.1 M) and 0.5 mL of culture extract was added and incubated at 37 ºC, 180 rpm, for 30 min. Reaction was stopped by the addition of 7.5 mL of acetone-ethanol-water mixture (1:1:1 volume fraction), and free fatty acids were titrated with NaOH 0.02 M. One unit of lipase activity (U.g-1 ) was considered to be the amount of enzyme that liberates 1 μmol of fatty acid per minute per gram of cultivated substrate (Burkert et al. 2004).
Lipase production test in aerated bioreactor
Reactions for the experimental design and validation were performed using 125 mL flasks, which are usually known as static flat-bed or tray reactors (Mitchell et al. 2006MITCHELL DA, KRIEGER N & BEROVIC M. 2006. Solid State Fermentation Bioreactors, Solid-State Fermentation Bioreactors: Fundamentals of Design and Operation. Springer, Heidelberg, 482 p.). To have a more comprehensive understanding of culture conditions upon lipase production, the determined optimal conditions in the static flat-bed were used in different types of reactors. This experiment was performed in bioreactors, in which the bed is static, but air is forcefully blown through the cultivation. For this, cylindrical glass flasks were used, with 28 cm high, 5.5 cm of diameter and sintered glass on the bottom. In this case, water-saturated air passed forcefully through the malt bagasse bed, at an air flow rate of 2 L.min-1, after passing through an air filter and distilled water to keep water saturation in aeration. For this experiment, 70 g of sterilized malt bagasse was used, keeping the same proportions of buffer and soybean oil defined by the CCD.
Lipase production test in pilot scale rotating drum bioreactor
To evaluate how this lipase production could behave under pilot scale conditions, a cultivation test was carried out in an rotating drum bioreactor, using 3 kg of non-autoclaved malt bagasse, keeping oil percentages and pH of buffer as obtained in the optimal conditions in the CCD analysis. The rotating drum bioreactor was equipped with circulating air above the bed to remove excess of metabolic gases, and agitation conditions were established as 30 min of 1 rpm agitation every 24 h of cultivation, before sample collection.
Solid enzymatic preparation
To obtain the Solid Enzymatic Preparation (SEP), malt bagasse was cultivated for 96 h under optimized conditions in cylindrical flasks with aeration through the bed, according to procedures described in the solid-state cultivation section. The cultivated malt bagasse was placed in a vacuum freeze dryer LGJ-12 lyophilizer for 24 h and stocked at -20 °C before use. The SEP obtained was tested according to section describing the lipase assay, using 0.5 g of SEP, to preliminarily evaluate possible practical uses of this product.
Analytical procedures
Lipid content was measured by Soxhlet direct extraction using petroleum ether as solvent in an automatic extractor SOXTEC Avanti 2055. Reducing sugars were measured by dinitrosalicylic acid method (DNS) and the results were determined in spectrophotometer (540 nm) (Miller et al. 1959). Ashes were determined according to literature (Silva & Queiroz 2002SILVA DJ & DE QUEIROZ AC. 2002. Análise de alimentos: métodos químicos e biológicos. UFV, Viçosa, 235 p.). Moisture content of malt bagasse was measured using a moisture analyzer (Ohaus MB32). Total protein content was measured using the Kjeldahl method, with conversion factor of 6.25 (Horwitz 1975HORWITZ. 1975. Official methods of analysis of the association of official analytical chemists, 12th edition. edited by William Horwitz. J Pharm Sci 65: 162.). Chemical characterization of spent malt (cellulose, hemicellulose, and lignin) was performed according to literature, using H2SO4 72 % for hydrolysis and sugar content was measured at HPLC with column HPX-87H at 45 °C, with 5 mM H2SO4 solution as mobile phase (Gouveia et al. 2009GOUVEIA ER, NASCIMENTO RT DO, SOUTO-MAIOR AM & ROCHA GJ DE M. 2009. Validação de metodologia para a caracterização química de bagaço de cana-de-açúcar. Quim Nova 32: 1500-1503.). During cultivation, lipase activity, soluble protein content, and pH were analyzed. Bradford method was used to measure soluble proteins, where Bradford reagent was added to the enzyme extract, and after 30 min incubation (20 oC), the content was read in a spectrophotometer (595 nm) (Zaia et al. 1998ZAIA DAM, ZAIA CTBV & LICHTIG J. 1998. Determinação de proteínas totais via espectrofometria: Vantagens e desvantagens dos métodos existentes. Quim Nova 21: 787-793.). For pH measurements, 5 g of total cultivated substrate was resuspended in 50 mL of distilled water, and allowed to rest for 15 min, under casual manual agitation. After 15 min, pH was obtained using a digital pH meter.
RESULTS AND DISCUSSION
Spent malt bagasse characterization
Results for the characterization of the spent malt bagasse used in this work are shown in Table II. Moisture values were similar to those found by Cordeiro et al. (2012)CORDEIRO LG, EL-AOUAR AA & GUSMÃO RP. 2012. Characterization of the bagasse coming from malt beer. Revista Verde 7: 20-22. with 75.5 % of water content in the biomass (Cordeiro et al. 2012CORDEIRO LG, EL-AOUAR AA & GUSMÃO RP. 2012. Characterization of the bagasse coming from malt beer. Revista Verde 7: 20-22.). Moisture is an important factor in SSC, with fungi needing low moisture content, usually around 40 to 60 %, thus, the malt bagasse was already appropriate for cultivation (Singhania et al. 2009SINGHANIA RR, PATEL AK, SOCCOL CR & PANDEY A. 2009. Recent advances in solid-state fermentation. Biochem Eng J 44: 13-18.). These water content values would, however, increase transportation costs and increase the risk of bacterial contamination (Cordeiro et al. 2012CORDEIRO LG, EL-AOUAR AA & GUSMÃO RP. 2012. Characterization of the bagasse coming from malt beer. Revista Verde 7: 20-22.) and therefore should be addressed in the industrial scale.
Lipid and ash contents (Table II) were similar to values found in the literature (Mello & Mali 2014MELLO LRPF & MALI S. 2014. Use of malt bagasse to produce biodegradable baked foams made from cassava starch. Ind Crops Prod 55: 187-193.). For lipase production, lipid content in malt bagasse may not be sufficient to induce enzyme activity, making necessary oil supplementation. In the production of lipase by Candida rugosa in SSC using rice bran as substrate, the most significant variables affecting enzyme production were found to be oil content, urea, and maltose (Venkata Rao et al. 1993VENKATA RAO P, JAYARAMAN K & LAKSHMANAN CM. 1993. Production of lipase by Candida rugosa in solid state fermentation. 1: determination of significant process variables. Process Biochem 28: 385-389.). Although studies showed that Aspergillus could produce extracellular lipase without lipid addition in substrate, adding oil as inducing agent can significantly improve lipase production. However, increasing the amount of lipid addition in cultivation does not necessarily correlate to higher lipase yields in Aspergillus cultivation (Ohnishi 1994OHNISHI K, YOSHIDA Y & SEKIGUCHI J. 1994. Lipase production of Aspergillus oryzae. J Ferment Bioeng 77: 490-495., Pokorny 1994).
Hemicellulose and lignin composition of the malt bagasse (Table II) were similar to values found by Mello et al. (2014) (23.41 % of hemicellulose and 26.13 % of lignin), and by Mussato et al. (2006) (28 % of hemicellulose and 28 % of lignin) (Mussatto et al. 2006MUSSATTO SI, DRAGONE G & ROBERTO IC. 2006. Brewers’ spent grain: Generation, characteristics and potential applications. J Cereal Sci 43: 1-14., Mello & Mali 2014MELLO LRPF & MALI S. 2014. Use of malt bagasse to produce biodegradable baked foams made from cassava starch. Ind Crops Prod 55: 187-193.). Cellulose, however, was higher than values reported by Mello et al. (2014) and Steinmacher et al. (2012)STEINMACHER NC, HONNA FA, GASPARETTO AV, ANIBAL D & GROSSMANN MVE. 2012. Bioconversion of brewer’s spent grains by reactive extrusion and their application in bread-making. LWT - Food Sci Technol 46: 542-547., (12.29 % and 11.35 %, respectively), which can be explained by malt bagasse composition varying based on barley varieties used, harvest time, conditions in malting of barley, and other conditions particular to each brewery (Santos et al. 2003SANTOS M, JIMÉNEZ JJ, BARTOLOMÉ B, GÓMEZ-CORDOVÉS C & DEL NOZAL MJ. 2003. Variability of brewer’s spent grain within a brewery. Food Chem 80: 17-21., Steinmacher et al. 2012STEINMACHER NC, HONNA FA, GASPARETTO AV, ANIBAL D & GROSSMANN MVE. 2012. Bioconversion of brewer’s spent grains by reactive extrusion and their application in bread-making. LWT - Food Sci Technol 46: 542-547.). Some filamentous fungi, such as Aspergillus, are good producers of cellulases, liberating sugars used in their metabolism (Liu et al. 2011LIU D, ZHANG R, YANG X, WU H, XU D, TANG Z & SHEN Q. 2011. Thermostable cellulase production of Aspergillus fumigatus Z5 under solid-state fermentation and its application in degradation of agricultural wastes. Int Biodeterior Biodegrad 65: 717-725.), thus the use of biomass with high cellulose content could be advantageous to reduce costs in nutritional supplementation in SSC using this microorganism.
CCD results
The experimental conditions and lipase activity values for 96 h (samples showing the highest activities in all experiments) of cultivation are shown in Table III. The response surface of variables pH, temperature, and percentage of oil are shown in Figure 1.
Response surfaces from CCD analysis of 96 h cultivation, of Aspergillus brasiliensis in malt bagasse, where (a) is influence of temperature and % of oil, (b) is influence of temperature and pH, and (c) is influence of % of oil and pH in lipase activity. Experiments were run using the static flat-bed bioreactor configuration.
CCD experimental conditions and lipase activity for 96 h cultivation showing standard deviation. Experiments were run using the static flat-bed bioreactor configuration.
The highest activity was 9.1 U.g-1, using phosphate buffer pH 7.0, 5 % of soybean oil and cultivation at 35 °C, whereas the lowest activity of 1.9 U.g-1 was obtained using acetate buffer at pH 4.7, 10 % of oil, and cultivation at 30 °C. Figure 2 presents the kinetics of lipase activity evolution during the CCD trails. Though we found higher lipase activity at 96 h of cultivation, some authors found longer times of cultivation time (120 h) for highest lipase activity in cultures of Aspergillus (Mahadik et al. 2002MAHADIK ND, PUNTAMBEKAR US, BASTAWDE KB, KHIRE JM & GOKHALE DV. 2002. Production of acidic lipase by Aspergillus niger in solid state fermentation. Process Biochem 38: 715-721., Colla et al. 2015COLLA LM, FICANHA AMM, RIZZARDI J, BERTOLIN TE, REINEHR CO & COSTA JAV. 2015. Production and characterization of lipases by two new isolates of Aspergillus through solid-state and submerged fermentation. Biomed Res Int 2015: 1-9.), but this difference is explained by the use of different cultivation conditions.
Kinetics of lipase activity during the CCD experiment for Apergillus brasiliensis 157f cultivation in the static flat-bed bioreactor configuration. Panels (a): treatments A (◼), B (◻), C (●), and D (○); (b): treatments E (▲), F (♦), G (▵), and H (◊); (c): treatments I (▼), J (▿), K (
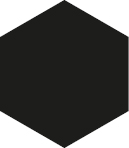
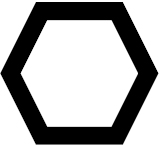
Analysis of the experimental data in Table III yielded the following second order equation (Eq. 1), relating the response variable (Y: lipase activity) to dependent factors (X1: pH, X2: % of soybean oil, and X3: temperature). In Table IV are presented optimal parameter values predicted by CCD analysis, and maximum predicted value of lipase activity in optimal conditions of cultivation was 8.2 U.g-1. Optimum pH and temperature obtained in this study for fungal lipase activity are similar to those already reported in literature, being around 30-40 °C and pH 6.0 to 8.0 (Colla et al. 2015COLLA LM, FICANHA AMM, RIZZARDI J, BERTOLIN TE, REINEHR CO & COSTA JAV. 2015. Production and characterization of lipases by two new isolates of Aspergillus through solid-state and submerged fermentation. Biomed Res Int 2015: 1-9., Cyndy et al. 2015CYNDY MF, ODACY CDS, MINELLI AS, ROBERTA C, OLIANE MCM, ERIKA VDM, KEILA AM & CRISTINA MDS-M. 2015. High-level lipase production by Aspergillus candidus URM 5611 under solid state fermentation (SSF) using waste from Siagrus coronata (Martius) Becari. African J Biotechnol 14: 820-828.).
Other studies also found pH value to be a contributing factor on lipase activity. For example, in the SSC of Rhodotorula glutini and C. rugosa in sugarcane bagasse and nutrient solution, highest lipase activity was achieved at pH range 7.0 to 8.0 (Ferrarezi et al. 2014FERRAREZI AL ET AL. 2014. Production and characterization of lipases and immobilization of whole cell of the thermophilic Thermomucor indicae seudaticae N31 for transesterification reaction. J Mol Catal B Enzym 107: 106-113.). The highest lipase activity in SSC of C. rugosa in groundnut oil cake was obtained at pH 6.0 (Rekha et al. 2012REKHA KSS, LAKSHMI MVVC, DEVI VS & KUMAR M. 2012. Production and Optimization of Lipase from Candida Rugosa Using Groundnut Oilcake Under Solid State. Int J Res Eng Technol 1: 571-577.).
As it is observed, highest lipase activity result was obtained at pH 7.0, using phosphate buffer. In Figure 3, it is possible to follow the variation of pH during the CCD experiment. It can be seen that those treatments with initial pH 6.00 or higher showed a decrease in pH at 48 h, stabilizing at a pH range of 3.0 to 4.0, coinciding with spore formation, indicating Aspergillus stress. In treatments with initial pH below 5.5, the formation of spores was not observed.
Kinetics of pH variation during the CCD experiment for Apergillus brasiliensis 157f cultivation in the static flat-bed bioreactor configuration. Panels (a): treatments A (◼), B (◻), C (●), and D (○); (b): treatments E (▲), F (▵), G (♦), and H (◊); (c): treatments I (▾), J (▿), K (
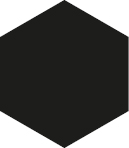
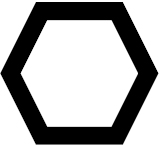
In this work, the variables oil percentage and temperature of cultivation were not found to be statistically significant, contrasting with other studies assessing lipase production by fungi in SSC (Treichel et al. 2010TREICHEL H, DE OLIVEIRA D, MAZUTTI MA, DI LUCCIO M & OLIVEIRA JV. 2010. A review on microbial lipases production. Food Bioprocess Technol 3: 182-196., Rekha et al. 2012REKHA KSS, LAKSHMI MVVC, DEVI VS & KUMAR M. 2012. Production and Optimization of Lipase from Candida Rugosa Using Groundnut Oilcake Under Solid State. Int J Res Eng Technol 1: 571-577.). Lipid content in the cultivation could be just an inducer, which corroborates with observed results in this work, in which higher percentages of oil were not paralleled with increased lipase production (Mahadik et al. 2002MAHADIK ND, PUNTAMBEKAR US, BASTAWDE KB, KHIRE JM & GOKHALE DV. 2002. Production of acidic lipase by Aspergillus niger in solid state fermentation. Process Biochem 38: 715-721.). In the present work, unlike most, no mineral salts were added to the medium in the SSC because we intended to produce lipase under the least expensive conditions. It has been demonstrated that some nutrients in culture medium might have significant influence in lipase production such as soybean meal and K2HPO4, as observed in cultures of Candida sp. in submerged fermentation (He & Tan 2006HE YQ & TAN TW. 2006. Use of response surface methodology to optimize culture medium for production of lipase with Candida sp. 99-125. J. Mol Catal B Enzym 43: 9-14.). Many reports on the use of agro-industrial residues for SSC used supplementations, such as NH4NO3, yeast extract, peptone, NH4Cl, and NaNO3, suggesting that one important factor is the C:N ratio (Kempka et al. 2008KEMPKA AP, LIPKE NL, DA LUZ FONTOURA PINHEIRO T, MENONCIN S, TREICHEL H, FREIRE DMG, LUCCIO MD & DE OLIVEIRA D. 2008. Response surface method to optimize the production and characterization of lipase from Penicillium verrucosum in solid-state fermentation. Bioprocess Biosyst Eng 31: 119-125., Mahanta et al. 2008MAHANTA N, GUPTA A & KHARE SK. 2008. Production of protease and lipase by solvent tolerant Pseudomonas aeruginosa PseA in solid-state fermentation using Jatropha curcas seed cake as substrate. Bioresour Technol 99: 1729-1735., Treichel et al. 2010TREICHEL H, DE OLIVEIRA D, MAZUTTI MA, DI LUCCIO M & OLIVEIRA JV. 2010. A review on microbial lipases production. Food Bioprocess Technol 3: 182-196.).
ANOVA results, in Table V, shows that the linear effect of pH was significant for lipase activity (p<0.05). The model prediction could explain 90.20 % of the lipase activity (R2 of 0.90), suggesting a good correlation between experimental results and theoretical values predicted by the model.
ANOVA results from the CCD analysis of Aspergillus brasiliensis bench scale SSC in malt bagasse.
Experimental validation
Using the optimized conditions obtained in statistical model (Table IV), a validating experiment was carried out in triplicate, with results shown in Figure 4. In 96 h, cultivation reached a maximum of 8.19 ± 0.34 U.g-1 of lipase activity, which is very similar to value predicted by the statistical model (8.17 U.g-1). Figure 4 also shows pH profile in cultivation, with similar behavior observed for treatments with initial pH of 6.0 or higher, showing a decrease of pH in 48 h with further stabilization in range of pH 3.0 to 4.0.
Profiles for lipase activity (◼) and pH (◻), during statistical validation of SSC of Aspergillus brasiliensis 157f in malt bagasse.
Lipase production test in bioreactors with fixed bed and forced aeration
Lipase production test in the plugged-flow bed with forced water-saturated aeration, had similar behavior as observed in the validation experiment using the static flat-bed conformation (Figure 5). Lipase activity at 96 h cultivation (9.83 U.g-1) was higher than found for the validation (8.19 U.g-1). Solid-state bioreactors with forced aeration trough fixed bed have better heat transfer rates and capacity to remove metabolic heat and CO2 from substrate (Mitchell et al. 2000MITCHELL DA, KRIEGER N, STUART DM & PANDEY A. 2000. New developments in solid-state fermentation II. Rational approaches to the design, operation and scale-up of bioreactors. Process Biochem 35: 1211-1225.). For instance, in SSC of P. decumbens in wheat straw and wheat bran to produce cellulases, highest enzyme productions were observed by using forced aeration in fixed bed configurations (Mo et al. 2004MO H, ZHANG X & LI Z. 2004. Control of gas phase for enhanced cellulase production by Penicillium decumbens in solid-state culture. Process Biochem 39: 1293-1297.).
Lipase activity (◼) and pH (◻) profiles of SSC of Aspergillus brasiliensis 157f in plugged-flow bed with forced water-saturated aeration bioreactors (malt bagasse).
Lipase production test in a pilot-scale rotating drum bioreactor with intermittent agitation
Lipase activity and pH results for rotating drum bioreactor with intermittent agitation (pilot-scale test) are shown in Figure 6. Lipase activity at 96 h (6.37 ± 0.02 U.g-1) was lower than results found for the other types of bioreactors tested. The pH profile had different behavior as observed in validation and cylindrical bioreactor with forced aeration. Lowest pH in this cultivation was 4.9. Mechanical mixing of the substrate was performed every 24 h for 30 min for homogenization. This agitation might have disrupted certain structures of the filamentous fungus, but could also facilitate cultivation in cases of strong bed packing problems (Mitchell et al. 2000MITCHELL DA, KRIEGER N, STUART DM & PANDEY A. 2000. New developments in solid-state fermentation II. Rational approaches to the design, operation and scale-up of bioreactors. Process Biochem 35: 1211-1225., 2006).
Lipase activity (◼) and pH (◻) profiles of SSC in the pilot-scale test in a rotating drum bioreactor of Aspergillus brasiliensis in malt bagasse.
Solid enzymatic preparation preliminary study
A possible strategy for reducing costs associated with the enzymatic route in biodiesel production is the use of a fermented solid, rich in lipase, produced by SSC, for the catalysis of reactions of esterification and transesterification (Salum et al. 2010SALUM TFC, VILLENEUVE P, BAREA B, YAMAMOTO CI, CÔCCO LC, MITCHELL DA & KRIEGER N. 2010. Synthesis of biodiesel in column fixed-bed bioreactor using the fermented solid produced by Burkholderia cepacia LTEB11. Process Biochem 45: 1348-1354.). In this case, not only the substrate would have low cost, using an agro-industrial waste, but also the costs of purification and immobilization of lipases would be reduced. To have a preliminarily evaluation of the possible use of the solid enzymatic preparation obtained after 96 h of SSC of A. brasiliensis 157f under the optimized parameters, we prepared a SEP of this material. Lipase activity measured in the SEP, after lyophilization, was 7.35 ± 0.13 U.g1. In this study, a preliminary test was used to evaluate final lipase activity in SEP and further studies are necessary to assess possible applications of this biocatalyst. This technique is interesting to be exploited because it avoids the need for expensive processes such as lipase purification and immobilization, having, for example, the potential to reduce costs in lipase-catalyzed biodiesel production (Salum et al. 2010SALUM TFC, VILLENEUVE P, BAREA B, YAMAMOTO CI, CÔCCO LC, MITCHELL DA & KRIEGER N. 2010. Synthesis of biodiesel in column fixed-bed bioreactor using the fermented solid produced by Burkholderia cepacia LTEB11. Process Biochem 45: 1348-1354.). This technique has been reported in literature for waste treatment and biodiesel production, as “Fermented Solid with Lipase Activity”, presenting a promising bio-product for industries (Rosa et al. 2006ROSA DR, CAMMAROTA MC, MARIA D & FREIRE G. 2006. Preparation Produced by Penicillium restrictum in Activated Oil and Grease 23: 814-823., Zago et al. 2014ZAGO E, BOTTON V, ALBERTON D, CÓRDOVA J, YAMAMOTO CI, CÔCCO LC, MITCHELL DA & KRIEGER N. 2014. Synthesis of ethylic esters for biodiesel purposes using lipases naturally immobilized in a fermented solid produced using Rhizopus microsporus. Energ Fuel 28: 5197-5203.). In effluent treatment tests with oil and grease, Rosa et al. (2006)ROSA DR, CAMMAROTA MC, MARIA D & FREIRE G. 2006. Preparation Produced by Penicillium restrictum in Activated Oil and Grease 23: 814-823. were able to use SEP with lipolytic activity of 32.3 U.g-1 produced in SSC of Penicillium restrictum using residues of the babassu oil industry as substrate, managing to make the effluent be under environmental legal disposal conditions. Such works represent an important contribution as an aid in biological treatment of effluents with high oil contents (Rosa et al. 2006ROSA DR, CAMMAROTA MC, MARIA D & FREIRE G. 2006. Preparation Produced by Penicillium restrictum in Activated Oil and Grease 23: 814-823.). In a similar work, producing lipase-rich SEP with R. microspores, Zago et al. (2014)ZAGO E, BOTTON V, ALBERTON D, CÓRDOVA J, YAMAMOTO CI, CÔCCO LC, MITCHELL DA & KRIEGER N. 2014. Synthesis of ethylic esters for biodiesel purposes using lipases naturally immobilized in a fermented solid produced using Rhizopus microsporus. Energ Fuel 28: 5197-5203. found high transesterification conversions of 68 % in 72 h of reaction time.
CONCLUSIONS
In this work, lipase production in solid-state cultivation in tray-type bioreactors (static flat-bed) was optimized using CCD analysis and validated. Under the same conditions, plugged-flow bed with forced water-saturated aeration bioreactors showed better results of lipase activity. These results demonstrated that forced aeration bioreactors could present better performances in industrial processes. Pilot-scale test in a rotating drum bioreactor showed lower lipase activity than observed for the other equipment, possibly because of fungi disruption. The solid enzymatic preparation showed lipase activity compatible with the previous cultivations and the lipolytic activity evaluated in the SEP and its low production costs justifies new researches for its possible application in industrial processes.
ACKNOWLEGMENTS
The authors wish to thank Conselho Nacional de Desenvolvimento Científico e Tecnológico (CNPq Brazil) and Coordenação de Aperfeiçoamento de Pessoal de Nível Superior (CAPES Brazil) for their financial support and scholarships.
REFERENCES
- ALIYU S & BALA M. 2013. Brewer’s spent grain: A review of its potentials and applications. African J Biotechnol 10: 324-331.
- ASHOK A, DORIYA K, RAO DRM & KUMAR DS. 2017. Design of solid state bioreactor for industrial applications: An overview to conventional bioreactors. Biocatal Agric Biotechnol 9: 11-18.
- BURKERT J. 2004. Optimization of extracellular lipase production by Geotrichum sp. using factorial design. Bioresour Technol 91: 77-84.
- CHAUHAN M, YENNAMALLI RM & GARLAPATI VK. 2016. Biochemical characterization and molecular modeling of a unique lipase from Staphylococcus arlettae JPBW-1. Eng Life Sci 16: 762-768.
- CHOUDHURY P & BHUNIA B. 2015. Industrial Application of Lipase: A Review. Biopharm J 1: 41-47.
- COLLA LM, FICANHA AMM, RIZZARDI J, BERTOLIN TE, REINEHR CO & COSTA JAV. 2015. Production and characterization of lipases by two new isolates of Aspergillus through solid-state and submerged fermentation. Biomed Res Int 2015: 1-9.
- CORDEIRO LG, EL-AOUAR AA & GUSMÃO RP. 2012. Characterization of the bagasse coming from malt beer. Revista Verde 7: 20-22.
- CONTESINI F, LOPES D, MACEDO G & NASCIMENTO M. 2010. Aspergillus sp. lipase: Potential biocatalyst for industrial use. J Mol Catal B Enzym 67: 163-171.
- CYNDY MF, ODACY CDS, MINELLI AS, ROBERTA C, OLIANE MCM, ERIKA VDM, KEILA AM & CRISTINA MDS-M. 2015. High-level lipase production by Aspergillus candidus URM 5611 under solid state fermentation (SSF) using waste from Siagrus coronata (Martius) Becari. African J Biotechnol 14: 820-828.
- DA SILVA MENEZES B, ROSSI DM & AYUB MAZ. 2017. Screening of filamentous fungi to produce xylanase and xylooligosaccharides in submerged and solid-state cultivations on rice husk, soybean hull, and spent malt as substrates. World J Microbiol Biotechnol 33: 58-72.
- DE CASTRO SM & DE CASTRO AM. 2012. Assessment of the Brazilian potential for the production of enzymes for biofuels from agroindustrial materials. Biomass Convers Biorefinery 2: 87-107.
- EDWINOLIVER NG, THIRUNAVUKARASU K, NAIDU RB, GOWTHAMAN MK, KAMBE TN & KAMINI NR. 2010. Scale up of a novel tri-substrate fermentation for enhanced production of Aspergillus niger lipase for tallow hydrolysis. Bioresour Technol 101: 6791-6796.
- FERRAREZI AL ET AL. 2014. Production and characterization of lipases and immobilization of whole cell of the thermophilic Thermomucor indicae seudaticae N31 for transesterification reaction. J Mol Catal B Enzym 107: 106-113.
- GOUVEIA ER, NASCIMENTO RT DO, SOUTO-MAIOR AM & ROCHA GJ DE M. 2009. Validação de metodologia para a caracterização química de bagaço de cana-de-açúcar. Quim Nova 32: 1500-1503.
- GUPTA R, KUMARI A, SYAL P & SINGH Y. 2015. Molecular and functional diversity of yeast and fungal lipases: Their role in biotechnology and cellular physiology. Prog Lipid Res 57: 40-54.
- HASAN F, SHAH AA & HAMEED A. 2006. Industrial applications of microbial lipases. Enzyme Microb Technol 39: 235-251.
- HE YQ & TAN TW. 2006. Use of response surface methodology to optimize culture medium for production of lipase with Candida sp. 99-125. J. Mol Catal B Enzym 43: 9-14.
- HORWITZ. 1975. Official methods of analysis of the association of official analytical chemists, 12th edition. edited by William Horwitz. J Pharm Sci 65: 162.
- KEMPKA AP, LIPKE NL, DA LUZ FONTOURA PINHEIRO T, MENONCIN S, TREICHEL H, FREIRE DMG, LUCCIO MD & DE OLIVEIRA D. 2008. Response surface method to optimize the production and characterization of lipase from Penicillium verrucosum in solid-state fermentation. Bioprocess Biosyst Eng 31: 119-125.
- KIRIN. 2017. Kirin Beer University Report Global Beer Production by Country in 2016. [accessed 2018 Aug 15]. http://www.kirinholdings.co.jp/english/news/2017/0810_01.html
» http://www.kirinholdings.co.jp/english/news/2017/0810_01.html - LIU D, ZHANG R, YANG X, WU H, XU D, TANG Z & SHEN Q. 2011. Thermostable cellulase production of Aspergillus fumigatus Z5 under solid-state fermentation and its application in degradation of agricultural wastes. Int Biodeterior Biodegrad 65: 717-725.
- MAHADIK ND, PUNTAMBEKAR US, BASTAWDE KB, KHIRE JM & GOKHALE DV. 2002. Production of acidic lipase by Aspergillus niger in solid state fermentation. Process Biochem 38: 715-721.
- MAHANTA N, GUPTA A & KHARE SK. 2008. Production of protease and lipase by solvent tolerant Pseudomonas aeruginosa PseA in solid-state fermentation using Jatropha curcas seed cake as substrate. Bioresour Technol 99: 1729-1735.
- MELLO LRPF & MALI S. 2014. Use of malt bagasse to produce biodegradable baked foams made from cassava starch. Ind Crops Prod 55: 187-193.
- MILLER GL. 1959. Use of Dinitrosalicylic Acid Reagent for Determination of Reducing Sugar. Anal Chem 31: 426-428.
- MITCHELL DA, KRIEGER N & BEROVIC M. 2006. Solid State Fermentation Bioreactors, Solid-State Fermentation Bioreactors: Fundamentals of Design and Operation. Springer, Heidelberg, 482 p.
- MITCHELL DA, KRIEGER N, STUART DM & PANDEY A. 2000. New developments in solid-state fermentation II. Rational approaches to the design, operation and scale-up of bioreactors. Process Biochem 35: 1211-1225.
- MO H, ZHANG X & LI Z. 2004. Control of gas phase for enhanced cellulase production by Penicillium decumbens in solid-state culture. Process Biochem 39: 1293-1297.
- MUSSATTO SI, DRAGONE G & ROBERTO IC. 2006. Brewers’ spent grain: Generation, characteristics and potential applications. J Cereal Sci 43: 1-14.
- OHNISHI K, YOSHIDA Y & SEKIGUCHI J. 1994. Lipase production of Aspergillus oryzae. J Ferment Bioeng 77: 490-495.
- POKORNY D, FRIEDRICH J & CIMERMAN A. 1994. Biotechnol Lett 16: 359-362.
- REKHA KSS, LAKSHMI MVVC, DEVI VS & KUMAR M. 2012. Production and Optimization of Lipase from Candida Rugosa Using Groundnut Oilcake Under Solid State. Int J Res Eng Technol 1: 571-577.
- ROSA DR, CAMMAROTA MC, MARIA D & FREIRE G. 2006. Preparation Produced by Penicillium restrictum in Activated Oil and Grease 23: 814-823.
- SALUM TFC, VILLENEUVE P, BAREA B, YAMAMOTO CI, CÔCCO LC, MITCHELL DA & KRIEGER N. 2010. Synthesis of biodiesel in column fixed-bed bioreactor using the fermented solid produced by Burkholderia cepacia LTEB11. Process Biochem 45: 1348-1354.
- SANTOS M, JIMÉNEZ JJ, BARTOLOMÉ B, GÓMEZ-CORDOVÉS C & DEL NOZAL MJ. 2003. Variability of brewer’s spent grain within a brewery. Food Chem 80: 17-21.
- SILVA DJ & DE QUEIROZ AC. 2002. Análise de alimentos: métodos químicos e biológicos. UFV, Viçosa, 235 p.
- SILVA WOB, MITIDIERI S, SCHRANK A & VAINSTEIN MH. 2005. Production and extraction of extracellular lipase from entomopathogenic fungus Metarhizium anisopliae (Clavicipitaceae: Hypocreales). Process Biochem 40: 321-326.
- SINGHANIA RR, PATEL AK, SOCCOL CR & PANDEY A. 2009. Recent advances in solid-state fermentation. Biochem Eng J 44: 13-18.
- STEINMACHER NC, HONNA FA, GASPARETTO AV, ANIBAL D & GROSSMANN MVE. 2012. Bioconversion of brewer’s spent grains by reactive extrusion and their application in bread-making. LWT - Food Sci Technol 46: 542-547.
- THIAGO R, PEDRO PM DE M & ELIANA FCS. 2014. Solid wastes in brewing process: A review. J Brew Distill 5: 1-9.
- THOMAS L, LARROCHE C & PANDEY A. 2013. Current developments in solid-state fermentation. Biochem Engineer J 81: 146-161.
- TREICHEL H, DE OLIVEIRA D, MAZUTTI MA, DI LUCCIO M & OLIVEIRA JV. 2010. A review on microbial lipases production. Food Bioprocess Technol 3: 182-196.
- VENKATA RAO P, JAYARAMAN K & LAKSHMANAN CM. 1993. Production of lipase by Candida rugosa in solid state fermentation. 1: determination of significant process variables. Process Biochem 28: 385-389.
- ZAGO E, BOTTON V, ALBERTON D, CÓRDOVA J, YAMAMOTO CI, CÔCCO LC, MITCHELL DA & KRIEGER N. 2014. Synthesis of ethylic esters for biodiesel purposes using lipases naturally immobilized in a fermented solid produced using Rhizopus microsporus. Energ Fuel 28: 5197-5203.
- ZAIA DAM, ZAIA CTBV & LICHTIG J. 1998. Determinação de proteínas totais via espectrofometria: Vantagens e desvantagens dos métodos existentes. Quim Nova 21: 787-793.
Publication Dates
-
Publication in this collection
12 Oct 2020 -
Date of issue
2020
History
-
Received
27 Aug 2018 -
Accepted
21 Nov 2018