Abstract
The effects of an aqueous extract of the plant Scoparia dulcis (200 mg/kg) on the polyol pathway and lipid peroxidation were examined in the liver of streptozotocin adult diabetic male albino Wistar rats. The diabetic control rats (N = 6) presented a significant increase in blood glucose, sorbitol dehydrogenase, glycosylated hemoglobin and lipid peroxidation markers such as thiobarbituric acid reactive substances (TBARS) and hydroperoxides, and a significant decrease in plasma insulin and antioxidant enzymes such as glutathione peroxidase (GPx), glutathione-S-transferase (GST) and reduced glutathione (GSH) compared to normal rats (N = 6). Scoparia dulcis plant extract (SPEt, 200 mg kg-1 day-1) and glibenclamide (600 µg kg-1 day-1), a reference drug, were administered by gavage for 6 weeks to diabetic rats (N = 6 for each group) and significantly reduced blood glucose, sorbitol dehydrogenase, glycosylated hemoglobin, TBARS, and hydroperoxides, and significantly increased plasma insulin, GPx, GST and GSH activities in liver. The effect of the SPEt was compared with that of glibenclamide. The effect of the extract may have been due to the decreased influx of glucose into the polyol pathway leading to increased activities of antioxidant enzymes and plasma insulin and decreased activity of sorbitol dehydrogenase. These results indicate that the SPEt was effective in attenuating hyperglycemia in rats and their susceptibility to oxygen free radicals.
Glutathione peroxidase; Glutathione-S-transferase; Insulin; Lipid peroxidation; Scoparia dulcis; Sorbitol dehydrogenase; Diabetes
Braz J Med Biol Res, April 2004, Volume 37(4) 577-586
Effect of an aqueous extract of Scoparia dulcis on blood glucose, plasma insulin and some polyol pathway enzymes in experimental rat diabetes
M. Latha and L. Pari
Department of Biochemistry, Faculty of Science, Annamalai University, Tamil Nadu, India
References
Correspondence and Footnotes
Correspondence and Footnotes
Correspondence and Footnotes
Abstract
The effects of an aqueous extract of the plant Scoparia dulcis (200 mg/kg) on the polyol pathway and lipid peroxidation were examined in the liver of streptozotocin adult diabetic male albino Wistar rats. The diabetic control rats (N = 6) presented a significant increase in blood glucose, sorbitol dehydrogenase, glycosylated hemoglobin and lipid peroxidation markers such as thiobarbituric acid reactive substances (TBARS) and hydroperoxides, and a significant decrease in plasma insulin and antioxidant enzymes such as glutathione peroxidase (GPx), glutathione-S-transferase (GST) and reduced glutathione (GSH) compared to normal rats (N = 6). Scoparia dulcis plant extract (SPEt, 200 mg kg-1 day-1) and glibenclamide (600 µg kg-1 day-1), a reference drug, were administered by gavage for 6 weeks to diabetic rats (N = 6 for each group) and significantly reduced blood glucose, sorbitol dehydrogenase, glycosylated hemoglobin, TBARS, and hydroperoxides, and significantly increased plasma insulin, GPx, GST and GSH activities in liver. The effect of the SPEt was compared with that of glibenclamide. The effect of the extract may have been due to the decreased influx of glucose into the polyol pathway leading to increased activities of antioxidant enzymes and plasma insulin and decreased activity of sorbitol dehydrogenase. These results indicate that the SPEt was effective in attenuating hyperglycemia in rats and their susceptibility to oxygen free radicals.
Key words: Glutathione peroxidase, Glutathione-S-transferase, Insulin, Lipid peroxidation, Scoparia dulcis, Sorbitol dehydrogenase, Diabetes
Introduction
Diabetes is likely to remain a significant threat to public health in the years to come. In the absence of effective and affordable interventions for both types of diabetes, the frequency of the disease will escalate worldwide, with a major impact on the population of developing countries (1). Hyperglycemia is an important factor in the development and progression of the complications of diabetes mellitus (2). The major metabolic changes caused by hyperglycemia are increased glucose flux into the polyol pathway, elevated formation of oxygen free radicals and formation of advanced glycated end products such as carboxymethyl lysine, a crystalline lens protein. Accumulation of glucose in red cells is said to enhance the irreversible production of glycosylated hemoglobin, which is a marker of red cell glycosylation in alloxan-diabetic rats (3). There is evidence that glycation itself may induce the formation of oxygen-derived free radicals in the erythrocytes of diabetic subjects. Glycosylated hemoglobin has been found to increase by about 16% in diabetic patients (4). Despite the considerable progress in the understanding and management of diabetes, the disease and the complications related to it continue to increase (5). Therefore, since diabetes is progressing unabated, there is an urgent need to identify indigenous natural sources of new active substances against the disease.
Scoparia dulcis (Scrophulariaceae), commonly known as sweet broomweed, is a perennial herb widely distributed in tropical and subtropical regions. In these regions, fresh or dried S. dulcis plants have been traditionally used as remedies for stomach troubles (6), hypertension (7), diabetes (8), bronchitis (9), and as analgesic and antipyretic agents (10). A number of different principles such as scoparic acid A, scoparic acid B, scopadulcic acid A and B, scopadulciol, and scopadulin (11) have been shown to contribute to the observed medicinal effect of the plant. These compounds were found to possess various biological activities such as inhibition of herpes simplex virus replication, gastric H+,K+-ATPase activation, antitumor activity, etc. Nath (12), in a study of the antidiabetic effect of S. dulcis, obtained a glycoside named ammelin from fresh plants which relieved other ailments accompanying diabetes, such as pyorrhea, eye troubles, joint pain, susceptibility to cold, etc., within a very short period of time.
To our knowledge, no reports are available on the effect of S. dulcis on the polyol pathway and lipid peroxidation. Thus, the main objectives of the present investigation were to ascertain the effect of an aqueous extract of S. dulcis on lipid peroxidation, the polyol pathway and the formation of glycosylated hemoglobin in rats with streptozotocin-induced diabetes.
Material and Methods
Experimental animals
Male albino Wistar rats weighing 180-200 g bred in the Central Animal House, Rajah Muthiah Medical College, Annamalai University, were used. The animals received a pellet diet (Hindustan Lever Ltd., Mumbai, India) and water ad libitum. The animals used in the present study were maintained in accordance with the guidelines of the National Institute of Nutrition, Indian Council of Medical Research, Hyderabad, India, and the study was approved by the Ethics Committee (Vide No. 73, 2002) of Annamalai University.
Drugs and chemicals
1-chloro-2,4-dinitrobenzene (CDNB), 5,5'-dithiobis-2-nitrobenzoic acid (DTNB), reduced glutathione (GSH), bovine serum albumin (BSA), thiobarbituric acid (TBA), nicotinamide adenine dinucleotide phosphate (NADPH), xylenol orange, and butylated hydroxy toluene (BHT) were obtained from Sigma (St. Louis, Mo, USA). All other chemicals used were obtained from local firms in India and were of the highest purity grade available.
Plant material
S. dulcis L. plants were collected from Neyveli, Cuddalore District, Tamil Nadu, India. The plant was identified and authenticated at the Herbarium of the Botany Directorate, Annamalai University. A voucher specimen (No. 3412) was deposited in the Botany Department of Annamalai University.
Preparation of the Scoparia dulcis aqueous extract
Five hundred grams of fresh whole S. dulcis plants was extracted with 1.5 liters of water by the method of continuous hot extraction and the filtrate was evaporated to constant weight in a rotavapor apparatus. The residual extract was dissolved in sterile water and used in the investigation (13).
Induction of experimental diabetes
Experimental animals received a freshly prepared solution of streptozotocin (45 mg/kg) in 0.1 M sodium citrate buffer, pH 4.5, injected intraperitoneally in a volume of 1 ml/kg (14). Control rats received 1 ml citrate buffer as vehicle. Forty-eight hours after streptozotocin administration, rats showing moderate diabetes with glycosuria and hyperglycemia (i.e., blood glucose levels of 200-300 mg/dl) were used for the experiment.
Experimental procedure
A total of 42 rats (30 surviving diabetic rats and 12 normal rats) were used in the experiment. The rats were divided into 7 groups of 6 animals each as follows: group 1, normal untreated rats; group 2, normal rats receiving the S. dulcis plant extract (SPEt, 200 mg/kg body weight) in aqueous solution daily by gavage for 6 weeks; group 3, diabetic control rats; group 4, diabetic rats receiving SPEt (50 mg/kg body weight) in aqueous solution daily by gavage for 6 weeks; group 5, diabetic rats receiving SPEt (100 mg/kg body weight) in aqueous solution daily by gavage for 6 weeks; group 6, diabetic rats receiving SPEt (200 mg/kg body weight) in aqueous solution daily by gavage for 6 weeks; group 7, diabetic rats receiving glibenclamide (600 µg/kg body weight) in aqueous solution daily by gavage for 6 weeks.
Blood samples were drawn at weekly intervals until the end of the 6-week study. At the end of the 6th week, all rats were killed by decapitation after anesthesia with pentobarbitone sodium (60 mg/kg). Blood was collected into tubes containing potassium oxalate and sodium fluoride solution for the estimation of blood glucose and plasma was separated for insulin assay. The liver was dissected out, washed in ice-cold saline, blotted dry, and weighed. The liver was selected because it is one of the tissues showing a high rate of free radical generation.
Biochemical analysis
Blood glucose and plasma insulin. Blood glucose was determined by the O-toluidine method (15). Plasma insulin was assayed by ELISA using a Boehringer-Mannheim kit with an ES300 Boehringer analyzer (Mannheim, Germany).
Oral glucose tolerance test. The test was carried out by the method of Du Vigneaud and Karr (16).
Hemoglobin and glycosylated hemoglobin. Hemoglobin was estimated by the method of Drabkin and Austin (17). Glycosylated hemoglobin (HbA1C) was estimated by the method of Sudhakar Nayak and Pattabiraman (18), modified by Bannon (19). Saline-washed red cells were treated with water for lysis and incubated at 37ºC for 15 min, and oxalate/HCl solution was then added and mixed. The filtrate was heated in a boiling water bath for 4 h, cooled with ice-cold water, treated with 40% TCA, and again centrifuged at 1000 g for 10 min. The supernatant obtained was then heated with 80% phenol and H2SO4 and the color developed was read at 480 nm after 30 min.
Lipid peroxidation. Lipid peroxidation in liver was estimated colorimetrically using TBARS and hydroperoxides by the method of Nichans and Samuelson (20) and Jiang et al. (21), respectively. Briefly, 0.1 ml of tissue homogenate (Tris-HCl buffer, pH 7.5) was treated with 2 ml of TBA-TCA-HCl (1:1:1) reagent (0.37% TBA, 0.25 N HCl and 15% TCA), placed in a water bath for 15 min, and cooled. The absorbance of the clear supernatant was measured against a reference blank at 535 nm.
The tissue homogenate (0.1 ml) was treated with 0.9 ml of Fox reagent (88 mg BHT, 7.6 mg xylenol orange and 9.8 mg ammonium ion sulfate added to 90 ml methanol and 10 ml 250 mM sulfuric acid) and incubated at 37ºC for 30 min. The color developed was read colorimetrically at 560 nm. Hydroperoxides are reported as mM/100 g tissue.
Sorbitol dehydrogenase assay. Sorbitol dehydrogenase, (SDH; EC. 1.1.1.14) was assayed by the method of Ulrich (22). An aliquot of liver homogenate was treated with 0.2 ml 12 mM and 1.6 ml 0.2 M triethanolamine buffer, pH 7.4. The reaction was started after 30 min by treatment with 0.3 ml 4 M D(-)fructose. Absorbance was determined with a Systronics UV-visible spectrophotometer (Dubai, UAE) at 60-s intervals for 5-8 min at 365 nm.
Glutathione peroxidase and reduced glutathione. Glutathione peroxidase (GPx) activity was measured by the method of Rotruck et al. (23). Briefly, the reaction mixture contained 0.2 ml 0.4 M sodium phosphate buffer, pH 7.0, 0.1 ml 10 mM sodium azide, 0.2 ml tissue homogenate (homogenized in 0.4 M sodium phosphate buffer, pH 7.0), 0.2 ml GSH, and 0.1 ml 0.2 mM hydrogen peroxide. The contents were incubated at 37ºC for 10 min, the reaction was stopped with 0.4 ml 10% TCA and centrifuged. The supernatant was assayed for GSH content using Ellman reagent (19.8 mg DTNB in 100 ml 0.1% sodium citrate).
Reduced GSH was determined by the method of Ellman (24). Briefly, 1.0 ml of the supernatant was treated with 0.5 ml Ellman reagent and 3.0 ml 0.2 M sodium phosphate buffer, pH 8.0, and absorbance was read at 412 nm. GSH peroxidase activity is reported as µg GSH consumed min-1 mg protein-1 and reduced GSH as mg/100 g tissue.
Glutathione-S-transferase (GST). The activity was determined spectrophotometrically by the method of Habig et al. (25). The reaction mixture (3 ml) contained 1.0 ml 100 mM sodium phosphate buffer, pH 6.5, 0.1 ml 30 mM CDNB and 1.7 ml twice-distilled water. After pre-incubating the reaction mixture at 37ºC for 5 min, the reaction was started by the addition of 0.1 ml tissue homogenate and 0.1 ml GSH as substrate and absorbance was monitored for 5 min at 340 nm. The reaction mixture without the enzyme was used as blank. GST activity is reported as µmol GSH-CDNB conjugate formed min-1 mg protein-1 using an extinction coefficient of 9.6 mM-1 cm-1.
Estimation of protein. Protein was determined by the method of Lowry et al. (26) using BSA as standard, at 660 nm.
Statistical analysis
Results are reported as means ± SD. Statistical analysis was performed by ANOVA followed by Duncan's multiple range test. P < 0.05 was taken to indicate a significant difference between groups (27).
Results
Blood glucose
Prior to streptozotocin administration, basal blood glucose levels did not differ significantly between groups, whereas 48 h after streptozotocin administration they were significantly higher in diabetic rats. Normal rats treated with SPEt were euglycemic throughout the course of the study.
Table 1 shows the effect of treatment with the extract on blood glucose levels. In the SPEt-treated groups (all doses), although a significant antihyperglycemic (P < 0.01) effect was evident from the first week onwards, the decrease in blood sugar was maximum by the end of the sixth week (65.63%; P < 0.001) in the group receiving 200 mg kg-1 day-1 SPEt. On the basis of these data, the dose 200 mg/kg per day SPEt was selected for further evaluation.
Effect of an aqueous extract of Scoparia dulcis on blood glucose, plasma insulin and some polyol pathway enzymes in
experimental rat diabetes. M. Latha and L. Pari. Brazilian Journal of Medical and Biological Research, 37 (4): 577, 2004.
[print this table in landscape]
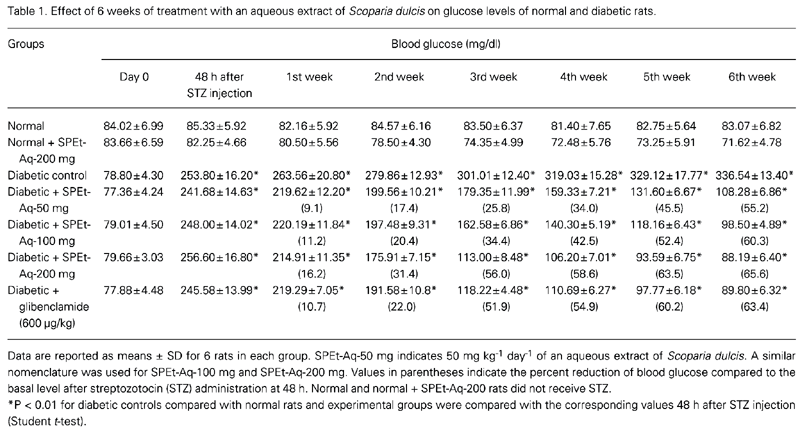
Oral glucose tolerance test
Table 2 presents the blood glucose levels of normal, diabetic control and SPEt- and glibenclamide-treated animals after oral administration of glucose (2 g kg-1 ml-1). In diabetic animals, blood glucose levels reached a peak 1 h after glucose administration. Although the glucose levels started to decline, they continued to be high after 2 h. SPEt-treated animals showed a significant decrease 1 and 2 h after oral glucose administration. Normal animals treated with SPEt showed a significant decrease in glucose levels at 120 min compared to 30 and 60 min.
Effect of an aqueous extract of Scoparia dulcis on blood glucose, plasma insulin and some polyol pathway enzymes in
experimental rat diabetes. M. Latha and L. Pari. Brazilian Journal of Medical and Biological Research, 37 (4): 577, 2004.
Plasma insulin, hemoglobin and HbA1C
Table 3 shows the plasma insulin, HbA1C and hemoglobin levels of normal and experimental animals. Administration of SPEt, 200 mg kg-1 day-1, and glibenclamide, 600 µg kg-1 day-1, to diabetic rats significantly increased plasma insulin and hemoglobin levels and significantly decreased HbA1C levels.
Effect of an aqueous extract of Scoparia dulcis on blood glucose, plasma insulin and some polyol pathway enzymes in
experimental rat diabetes. M. Latha and L. Pari. Brazilian Journal of Medical and Biological Research, 37 (4): 577, 2004.
Sorbitol dehydrogenase, lipid peroxidation and antioxidants
Table 4 presents the SDH, GPx, GST, and GSH activities and the TBARS and hydroperoxide levels in the liver of normal and experimental rats. SDH activity and TBARS and hydroperoxide levels were significantly increased whereas GPX, GST and GSH were significantly decreased in diabetic rats. Administration of SPEt and glibenclamide decreased the SDH activity and lipid peroxidation products and increased the enzymatic antioxidants in the liver of diabetic rats. Normal rats treated with SPEt did not show any significant alteration. The effect of SPEt was comparable to that of glibenclamide.
Effect of an aqueous extract of Scoparia dulcis on blood glucose, plasma insulin and some polyol pathway enzymes in
experimental rat diabetes. M. Latha and L. Pari. Brazilian Journal of Medical and Biological Research, 37 (4): 577, 2004.
Discussion
The increasing use of medicinal plants is due to the extraction and development of several successful drugs and chemotherapeutic agents from plants as well as their use as traditional rural herbal remedies (5). Streptozotocin-induced hyperglycemia in rodents is considered to be a good model for the preliminary screening of agents active against type II diabetes (28) and is widely used. Streptozotocin, N-{methylnitrocarbamoyl}-D-glucosamine, is a potent DNA methylating agent and acts as a nitric oxide donor in pancreatic cells. ß-cells are particularly sensitive to damage by nitric oxide and free radicals because of their low levels of free radical scavenging enzymes (29). In the present investigation, treatment with SPEt showed a significant antihyperglycemic activity. The maximum reduction in glucose levels was observed in groups receiving 200 mg/kg of the extract. Although the mechanism of action of the SPEt extract is unknown, a number of other plants have been reported to have antihyperglycemic and insulin release-stimulating effects (30,31) or to contain insulin (32,33).
SPEt might enhance glucose utilization since it significantly reduces blood glucose in diabetic rats. From the data obtained with the oral glucose tolerance test, it is clear that blood glucose levels reached a peak and returned to fasting values after 2 h in both normal and treated rats (diabetic + SPEt and diabetic + glibenclamide). Elevated blood glucose levels remained high even after 2 h in diabetic rats. SPEt administration effectively prevented the increase in blood glucose without causing a hypoglycemic state, an effect due to the restoration of the delayed insulin response. In this context, other medicinal plants such as Cassia auriculata and phaseolus vulgaris have also been reported to have the same effect (30,31). Administration of SPEt to normal animals also produced a significant reduction in blood glucose levels.
In uncontrolled or poorly controlled diabetes there is increased glycosylation of a number of proteins including hemoglobin and the a-crystalline protein of the lens. In long-term diabetes the glycosylated form of hemoglobin shows altered affinity for oxygen, which may be a factor in tissue anoxia (34).
In diabetes, the glycation and subsequent browning (glycoxidation) reactions are enhanced by elevated glucose levels and there is some evidence that glycation itself may induce the formation of oxygen-derived free radicals (4). Studies have shown that HbA1C comprises 3.4 to 5.8% of total hemoglobin in normal red cells, but is elevated in patients with diabetes mellitus (35). HbA1C levels are monitored as a reliable index of glycemic control in diabetes (36). In the current study, HbA1C levels followed a similar pattern, i.e., they were elevated in diabetic rats and the extent of this increase was directly proportional to fasting blood glucose levels (37). Koenig et al. (36) also reported a 16% increase in HbA1C levels in diabetic patients. Total hemoglobin decreased in the diabetic group, possibly due to the increased formation of HbA1C. This result was well correlated with an earlier report of decreased hemoglobin levels in experimentally diabetic rats (38). The increase in hemoglobin levels in animals receiving SPEt may have been due to the decreased blood glucose levels. In this context, several medicinal plants have also been reported to have the ability to reduce HbA1C levels in diabetic rats (31).
The conversion of glucose to sorbitol, which is catalyzed by aldose reductase (EC 1.1.1.21) in the presence of NADPH, may be related to the protection against oxidative stress and to abnormalities in nitric oxide action (39). NADPH levels are reduced by elevated polyol pathway flux, impairing the GSH redox cycle (4), which is an important mechanism of cell protection against oxygen-derived free radicals. The oxygen-derived free radicals are markedly increased in diabetes and, if not scavenged, they cause damage to the vascular endothelium and neutralize nitric oxide (4). Malondialdehyde, which is a secondary product of lipid peroxidation, is known to cause cross-linkage of membrane components containing amino groups which makes the membrane fragile (40). In agreement with the above findings, we observed a significant increase in lipid peroxidation products (TBARS and hydroperoxides) in streptozotocin diabetic rats. This was significantly attenuated by treatment with SPEt since S. dulcis has been reported to be rich in flavonoids and diterpenoids, well-known antioxidants (11) that scavenge the free radicals generated during diabetes.
SDH catalyzes the conversion of sorbitol to fructose in the presence of NAD. SDH activity has been reported to be elevated in diabetic rats, leading to increased fructose availability, with fructose being a 10-fold better substrate than glucose for glycosylation (41). In the present study, an increase in SDH activity was observed in the liver of diabetic rats. Since plasma and liver glucose concentrations are increased in diabetic rats, more glucose is converted to sorbitol. The elevation in SDH activity observed in diabetic rats may have been due to the increased availability of sorbitol. SDH activity was found to be significantly reduced after treatment with SPEt.
The pathophysiological consequences of GSH depletion have been extensively studied. GSH, GPx and GST depletion promotes generation of reactive oxygen species and oxidative stress with the subsequent cascade of effects affecting the functional and structural integrity of cell and organelle membranes (42). Treatment with SPEt increased GSH content. High GSH levels protect cellular proteins against oxidation through the GSH redox cycle and also directly detoxify the reactive oxygen species generation induced by exposure to streptozotocin (42).
S. dulcis lowers blood glucose in diabetic rats, with a consequent increase in the NADPH/NADP ratio resulting in increased GSH reductase activity. This, in turn, elevates the availability of GSH, the substrate for GPx, with an increase in GPx activity, which in turn scavenges H2O2. The entire process can be explained by a mechanism whereby increased flux through the polyol pathway reduces the effectiveness of the GSH redox cycle in scavenging free radicals (Figure 1).
Thus, the present results demonstrate that S. dulcis may play a protective role in diabetes mellitus. Administration of SPEt to diabetic rats appeared to attenuate hyperglycemia and their susceptibility to oxygen-derived free radicals by reducing the influx of glucose through the polyol pathway, thus maintaining GSH at optimal concentrations.
Address for correspondence: L. Pari, Department of Biochemistry, Faculty of Science, Annamalai University, Annamalai Nagar-608 002, Tamil Nadu, India. Fax: +91-4144-23-8145. E-mail: paribala@sancharnet.in
Research supported by University Grants Commission, New Delhi (Project No. F.12-36/2001, SR-I) to L. Pari and a research fellowship to M. Latha. Received June 24, 2003. Accepted January 16, 2004.
- 1. Marx J (2002). Unravelling the causes of diabetes. Science, 296: 686-689.
- 2. Luzi L (1998). Pancreas transplantation and diabetic complications. New England Journal of Medicine, 339: 115-117.
- 3. Al-Shamaony LA, Al-Khazraji SM & Twaiji HAA (1994). Hypoglycemic effect of Artemisia herba alba II. Effect of a valuable extract on some blood parameters in diabetic animals. Journal of Ethnopharmacology, 43: 167-171.
- 4. Inouye M, Hashimoto H, Mio T & Sumino K (1998). Levels of lipid peroxidation product and glycated hemoglobin A1C in the erythrocytes of diabetic patients. Clinica Chimica Acta, 276: 163-172.
- 5. Tiwari AK & Madhusudana Rao J (2002). Diabetes mellitus and multiple therapeutic approaches of phytochemicals: present status and future prospects. Current Science, 83: 30-38.
- 6. Satyanarayana K (1969). Chemical examination of Scoparia dulcis (Linn): Part I. Journal of the Indian Chemical Society, 46: 765-766.
- 7. Chow SY, Chen SM, Yang CM & Hsu H (1974). Pharmacological studies on China herbs (I). Hypotensive effect of 30 Chinese herbs. Journal of the Formosan Medical Association, 73: 729-739.
- 8. Perry LM (1980). Medicinal Plants of East and Southeastasia: Attributed Properties and Uses The MIT Press, Cambridge, MA, USA.
- 9. Gonzales Torres DM (1986). Catalogo de Plantas Medicinales (y Alimenticias y Utiles) Usada en Paraguay Asunción, Paraguay.
- 10. Farias Freie SM, Silva Emin JA, Lapa AJ, Souccar C & Brandao Torres LM (1993). Analgesic and anti-inflammatory properties of Scoparia dulcis L. extract and glutinol in rodents. Phytotherapy Research, 7: 408-414.
- 11. Hayashi T, Kawaski M, Miwa Y, Taga T & Morita N (1990). Antiviral agents of plant origin III. Scopadulin, a novel tetracyclic diterpene from Scoparia dulcis L. Chemical and Pharmaceutical Bulletin, 38: 945-947.
- 12. Nath MC (1943). Investigations on the new antidiabetic principle (amellin) occurring in nature. Part I. Studies on some of its biochemical properties. Annals of Biochemistry and Experimental Medicine, 3: 55-62.
- 13. Jain SR (1968). Hypoglycemic principle in the Musa sapientum and its isolation. Planta Medica, 1: 43-47.
- 14. Siddique O, Sun Y, Lin JC & Chien YW (1987). Facilitated transdermal transport of insulin. Journal of Pharmaceutical Sciences, 76: 341-345.
- 15. Sasaki T, Masty S & Sonae A (1972). Effect of acetic acid concentration on the color reaction in the O-toluidine boric acid method for blood glucose estimation. Rinshbo Kagaku, 1: 346-353.
- 16. Du Vigneaud V & Karr WG (1925). Carbohydrate utilization rate of disappearance of D-glucose from the blood. Journal of Biological Chemistry, 66: 281-300.
- 17. Drabkin DL & Austin JM (1932). Spectrophotometric constants for common hemoglobin derivatives in human dog and rabbit blood. Journal of Biological Chemistry, 98: 719-733.
- 18. Sudhakar Nayak S & Pattabiraman T (1982). A new colorimetric method for the estimation of glycosylated hemoglobin. Clinica Chimica Acta, 109: 267-274.
- 19. Bannon P (1982). Effect of pH on the elimination of the labile fraction of glycosylated hemoglobin. Clinical Chemistry, 28: 2183.
- 20. Nichans WG & Samuelson D (1968). Formation of malondialdehyde from phospholipid arachidonate during microsomal lipid peroxidation. European Journal of Biochemistry, 6: 126-130.
- 21. Jiang ZY, Hunt JV & Wolff SP (1992). Ferrous ion oxidation in the presence of xylenol orange for detection of lipid hydroperoxide in low-density lipoprotein. Analytical Biochemistry, 202: 384-387.
- 22. Ulrich HB (1974). Methods of Enzymatic Analysis Vol. 2. Edited by HU Bergmeyer, 2nd English edition. Verlag Chemie Weinheim. Academic Press Inc., New York, San Francisco and London, 569-573.
- 23. Rotruck JT, Pope AL, Ganther HE & Swanson AB (1984). Selenium: Biochemical roles as a component of glutathione peroxidase. Science, 179: 588-590.
- 24. Ellman GL (1959). Tissue sulfhydryl groups. Archives of Biochemistry and Biophysics, 82: 70-77.
- 25. Habig WR, Pbst MJ & Jakpoly WB (1974). Glutathione transferase. A first enzymatic step in mercapturic acid formation. Journal of Biological Chemistry, 249: 7130-7139.
- 26. Lowry OH, Rosebrough MJ, Farr AL & Randall RJ (1951). Protein measurement with the Folin phenol reagent. Journal of Biological Chemistry, 193: 265-275.
- 27. Duncan BD (1957). Multiple range tests for correlated and heteroscedastic means. Biometrics, 13: 359-364.
- 28. Ivorra MD, Paya M & Villar A (1989). A review of natural products and plants as potential antidiabetic drugs. Journal of Ethnopharmacology, 27: 243-275.
- 29. Spinas GA (1999). The dual role of nitric oxide in islet ß-cells. News in Physiological Sciences, 14: 49-54.
- 30. Latha M & Pari L (2003). Preventive effects of Cassia auriculata L. flowers on brain lipid peroxidation in rats treated with streptozotocin. Molecular and Cellular Biochemistry, 243: 23-28.
- 31. Venkateswaran S & Pari L (2002). Antioxidant effect of phaseolus vulgaris in streptozotocin-induced diabetic rats. Asia Pacific Journal of Clinical Nutrition, 11: 206-209.
- 32. Silva LB, Santos SSS, Azevedo CR et al. (2002). The leaves of green plants as well as a cyanobacterium, a red alga, and fungi contain insulin-like antigens. Brazilian Journal of Medical and Biological Research, 35: 297-303.
- 33. Oliveira AEA, Machado OLT, Gomes VM, Xavier Neto J, Pereira AC, Vieira JGH, Fernandes KVS & Xavier-Filho J (1999). Jack bean seed coat contains a protein with complete sequence homology to bovine insulin. Protein and Peptide Letters, 6: 15-21.
- 34. Bunn HF, Shapiro R, McManns M, Garrick L, MacDonald HH, Gallop PM & Gabby KH (1979). Structural heterogenicity of human hemoglobin A due to non-enzymic glycosylation. Journal of Biological Chemistry, 254: 3892-3898.
- 35. Trivelli LA, Ranney HM & Lai IIT (1971). Hemoglobin components in patients with diabetes mellitus. New England Journal of Medicine, 284: 353-357.
- 36. Koenig RJ, Peterson CM, Jones RL, Saudek C, Lehrman M & Cerami A (1978). Correlation of glucose regulation and hemoglobin AIc in diabetes mellitus. New England Journal of Medicine, 295: 417-420.
- 37. Jackson R, Hers RL & England JD (1979). HbA1c values in children with overt diabetes maintained in varying degree of control. Diabetes care, 2: 391-395.
- 38. Venkateswaran S & Pari L (2002). Effect of Coccinia indica on blood glucose, insulin and hepatic key enzymes in experimental diabetes. Pharmaceutical Biology, 40: 165-170.
- 39. Fredgey K (1995). Ten-year retrospective on the antioxidant hypothesis of arteriosclerosis: Threshold plasma levels of antioxidant micronutrients related to minimum cardiovascular risk. Journal of Nutritional Biochemistry, 6: 206-236.
- 40. Cameron NE & Cotter MA (1994). The relationship of vascular changes to metabolic factors in diabetes mellitus and their role in the development of peripheral nerve complications. Diabetes/Metabolism Reviews, 10: 189-224.
- 41. Brownlee M (1992). Glycation products and the pathogenesis of diabetic complications. Diabetes Care, 15: 1835-1843.
- 42. Raza H, Ahmed I, John A & Sharma AK (2000). Modulation of xenobiotic metabolism and oxidative stress in chronic streptozotocin induced diabetic rats fed with Momordica charantia fruit extract. Journal of Biochemistry and Molecular Toxicology, 3: 131-139.
Correspondence and Footnotes
Publication Dates
-
Publication in this collection
22 Apr 2004 -
Date of issue
Apr 2004
History
-
Received
24 June 2003 -
Accepted
16 Jan 2004