Abstract
The present study analyzes Na+ and K+ disturbances caused by low pH in two catfish species from the Amazon River. Corydoras adolfoi inhabits ion-poor, black-stained, low pH (3.5-4.0) waters, while C. schwartzi is native to ion-rich waters at circumneutral pH. Fish were exposed to pH 3.5 Ca2+-free, and Ca2+-enriched (~500 µmol/l) water to determine the protective effects of calcium. Net Na+ and K+ fluxes were measured in the water collected from the fish experimental chambers. C. adolfoi was unable to control the Na+ efflux at low pH, exhibiting Na+ loss up to -594 ± 84 nmol g-1 h-1 during the first hour. After 3 and 6 h, net Na+ flux increased by 7- and 23-fold, respectively. In C. schwartzi, at pH 3.5, the initial high Na+ loss (-1,063 ± 73 nmol g-1 h-1) was gradually attenuated. A K+ loss occurred in both species, but remained relatively constant throughout exposure. High [Ca2+] affected ion losses in both species. C. adolfoi had 70% loss attenuation, indicating incapacity to control Na+ efflux. In C. schwartzi, elevated [Ca2+] completely prevented the Na+ losses caused by exposure to low pH. Rather different patterns were seen for K+ fluxes, with C. adolfoi showing no K+ disruption when exposed to low pH/high [Ca2+]. Thus, C. adolfoi loses Na+ during acid exposure, but has the ability to control K+ loss, while C. schwartzi controls diffusive Na+ loss but exhibits a slightly higher K+ loss. Ion balance was influenced by [Ca2+] at low pH in C. schwartzi but not in C. adolfoi.
Low pH; Calcium; Blackwater; Net Na+ and K+ flux; Amazon; Catfish
Braz J Med Biol Res, March 2002, Volume 35(3) 361-367
Low pH and calcium effects on net Na + and K + fluxes in two catfish species from the Amazon River ( Corydoras : Callichthyidae)
A.Y. Matsuo and A.L. Val
Laboratório de Ecofisiologia e Evolução Molecular, Instituto Nacional de Pesquisa da Amazônia, Manaus, AM, Brasil
References
Correspondence and Footnotes Correspondence and Footnotes Correspondence and Footnotes
Abstract
The present study analyzes Na+ and K+ disturbances caused by low pH in two catfish species from the Amazon River. Corydoras adolfoi inhabits ion-poor, black-stained, low pH (3.5-4.0) waters, while C. schwartzi is native to ion-rich waters at circumneutral pH. Fish were exposed to pH 3.5 Ca2+-free, and Ca2+-enriched (~500 µmol/l) water to determine the protective effects of calcium. Net Na+ and K+ fluxes were measured in the water collected from the fish experimental chambers. C. adolfoi was unable to control the Na+ efflux at low pH, exhibiting Na+ loss up to -594 ± 84 nmol g-1 h-1 during the first hour. After 3 and 6 h, net Na+ flux increased by 7- and 23-fold, respectively. In C. schwartzi, at pH 3.5, the initial high Na+ loss (-1,063 ± 73 nmol g-1 h-1) was gradually attenuated. A K+ loss occurred in both species, but remained relatively constant throughout exposure. High [Ca2+] affected ion losses in both species. C. adolfoi had 70% loss attenuation, indicating incapacity to control Na+ efflux. In C. schwartzi, elevated [Ca2+] completely prevented the Na+ losses caused by exposure to low pH. Rather different patterns were seen for K+ fluxes, with C. adolfoi showing no K+ disruption when exposed to low pH/high [Ca2+]. Thus, C. adolfoi loses Na+ during acid exposure, but has the ability to control K+ loss, while C. schwartzi controls diffusive Na+ loss but exhibits a slightly higher K+ loss. Ion balance was influenced by [Ca2+] at low pH in C. schwartzi but not in C. adolfoi.
Key words: Low pH, Calcium, Blackwater, Net Na+ and K+ flux, Amazon, Catfish
Introduction
The Amazon blackwaters are known by their singular physicochemical features, which are unusual relative to the world average values for freshwater (1). The two most noticeable parameters are low pH and low ion concentration. The Rio Negro River, for instance, presents pH levels around 4.5-6.0, which are as low as 3.0 in restricted flooded forest areas (the igapós) (2). The Amazon nutrient-rich waters, also called whitewaters, drain from the Andean and pre-Andean regions, and their pH is usually around circumneutral values (1). In spite of such extremely adverse features for aquatic living organisms, blackwater habitats are not as inhospitable as one would expect. Fish diversity for the Rio Negro River was estimated at more than 1000 species (3). How these specimens can thrive in such conditions and what the adaptive strategies developed by them are still poorly understood.
Acid waters with low ion concentrations such as found in blackwaters may induce severe ion disruption in fish, including K+ losses from the epithelial cells themselves due to the toxic effects triggered by high [H+] (4). These effects can be summarized as the inhibition of ion uptake and an elevation of ion efflux (5). Calcium has long been known to reduce the toxicity of acid waters to fish (6-10). Apparently, there is a Ca2+ versus H+ competition for sites on tight junctions that maintain epithelial integrity, as well as evidence that bound Ca2+ shields access to Na+-binding sites on the apical transporters (5). Thus, high [H+] displaces Ca2+ from the binding sites and diffusive losses take place (4).
Corydorasadolfoi Burgess, 1982, is a catfish commonly inhabiting blackwaters in the flooded forests of the middle Rio Negro River, withstanding pH's around 3.5~4.0 (11). C. schwartzi Rössel, 1963, was used for comparison because it is a species endemic to whitewaters of the Purus River, living at circumneutral pH. The aim of the present study was to evaluate the disturbances in the net Na+ and K+ fluxes in these catfish from the Amazon region exposed to acute low pH, in an attempt to assess how they can deal with low pH. The possible protective influence of Ca2+ was also analyzed.
Material and Methods
Experimental fish
Fish collected from their native environments were obtained from ornamental fish exporters (Corydoras Tetra Aquarium and Turky's Aquarium) in the municipality of Manaus, Amazon, Brazil. They were acclimated for at least 14 days before the beginning of the experiments at the Laboratory of Ecophysiology and Molecular Evolution of INPA. Fish were held in 60-liter aquaria, provided with pump aerators, carbon-zeolite filters, and supplied with INPA ground water (average concentrations: 15 µmol/l Na+, 16 µmol/l Cl-, 9 µmol/l K+, 9 µmol/l Ca2+, and 2.05 mg/l dissolved organic carbon; see Ref. 12). Ground water pH was 6.0 ± 0.5 after two days of aeration to eliminate excess CO2 before use. Water temperature was 27 ± 2ºC. All fish weighed 1.5 to 3.5 g. The specimens were fed commercial dried pellets daily, and food was withdrawn 24 h prior to experimentation.
Experimental protocol
Ten fish were used in each treatment, including a control group. Aerated ground water was used as the `standard water'. Experimental chambers consisted of 200-ml polyethylene darkened chambers; small holes on the lid were used to supply water (recirculating system) and aeration, and for pH adjustments. The fish were placed in these chambers 12 h before starting the experiments. At the beginning of the experiment, the flow was stopped, and water in the chambers was totally replaced with fresh standard water. A 10-ml water sample was taken and a second sample was taken from each chamber 1 h later (control, pH 6.0). This procedure was performed for all fish of all treatments to confirm fish homeostasis and any possible disturbance due to the transfer to chambers. Water samples were stored in plastic vials and refrigerated until subsequent analysis for Na+ and K+ concentrations.
Low pH treatment
After sampling during the control period, standard water in the chamber was completely changed by emptying it manually and replacing with experimental solution. Acid solution (pH 3.5 ± 0.2) was previously prepared by the addition of 1 N H2SO4 to standard water, and kept aerated in a plastic box for 12 h before use. Calcium concentration in the solution was about 9 µmol/l (Ca2+ from the ground water). The pH inside the chambers was periodically checked using a portable pH meter and adjusted as necessary by the addition of diluted H2SO4. Water samples (10 ml) were taken 1, 3, and 6 h after the water was changed. Acid solution was then replaced with standard water again, and the recovery period was started. Samples in the recovery series were taken after 1 and 3 h. Fish were weighed and then released into a holding aquarium. An identical control series was performed for each species with the same water changeovers, but using pH 6.0 throughout.
Effects of calcium at low pH
The experimental protocol for this treatment was similar to that described before, except that CaCl2 was added to obtain a ~500 µmol/l solution. The standard water was replaced with this solution after first sampling, which in turn was replaced with standard water after 6 h. Water samples were taken as previously described.
Net Na + and K + flux measurements
A flame photometer (CELM FC-180 model) calibrated with known standards was used to obtain Na+ and K+ readings in the water. Net Na+ and K+ flux measurements were made according to the following equation (13):
Jnet = ([ion1] - [ion2]) . V . (M . t)-1,
where [ion1] and [ion2] are the ion concentrations (nmol/l) at the beginning and at the end of the flux period, respectively; V is the water volume in the chamber (liters); M is the fish weight (g), and t is the duration of the flux period (h).
Statistical analysis
All data are reported as means ± SEM. Mean values of net ion fluxes in the treatments were compared to the control groups by one-way ANOVA (P<0.05). When significant differences were detected, Dunnett's multiple comparison test was applied.
Results
All fish were in approximate homeostasis during the control pretreatment phase, with very small net ion losses to the water. Jnet values for Na+ and K+ were not significantly different between the two species at the beginning of the treatments. Net ion fluxes were about -189 ± 22 (Na+) and -56 ± 14 nmol g-1 h-1 (K+) for C. adolfoi during the pretreatment period. The control values for C. schwartzi net fluxes were -252 ± 50 (Na+) and -264 ± 52 nmol g-1 h-1 (K+). Fish had acclimated to the chambers and had recovered from handling procedures during the transfer.
Net fluxes at pH 3.5
C. adolfoi, the blackwater species, had an overall Na+ loss about 10-fold higher than that of C. schwartzi, the whitewater species, at pH 3.5, showing large and significant differences relative to the control treatment (Figure 1). In the first hour of exposure to pH 3.5, C. adolfoi exhibited a 2-fold increase in Na+ loss (to -594 ± 84 nmol g-1 h-1). After 3 and 6 h, Jnet Na+ increased significantly by as much as 7- and 23-fold (to -4,670 ± 371 and -14,171 ± 1,053 nmol g-1 h-1), respectively, indicating that C. adolfoi was unable to control Na+ efflux under acute low pH. No deaths occurred during this treatment, and efflux was quickly reduced during the recovery period.
C. schwartzi showed the capacity to withstand pH 3.5 fairly well (Figure 2). Although there was an initially high efflux during the first hour, when Jnet Na+ was about 3 times higher (-1,063 ± 73 nmol g-1 h-1) than control, the losses were gradually attenuated after 3 and 6 h (-561 ± 44 and -364 ± 33 nmol g-1 h-1, respectively), exactly the opposite pattern to that seen in C. adolfoi. In the recovery series, net Na+ flux decreased significantly as well (-112 ± 26 and -35 ± 13 nmol g-1 h-1, respectively).
Significant K+ loss occurred in both species (Figures 3 and 4). For C. adolfoi, the average loss was about 3 times higher than the control treatment (-200 ± 22 nmol g-1 h-1), and remained constant throughout exposure. This feature suggests that the fish were able to control diffusive efflux. C. schwartzi exhibited a pronounced K+ loss in the first hour of treatment (-419 ± 11 nmol g-1 h-1), with some later attenuation. After continued exposure, these K+ effluxes were -241 ± 13 and -201 ± 9 nmol g-1 h-1, at 3 and 6 h, respectively.
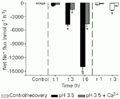
[View larger version of this image (7 K GIF file)]
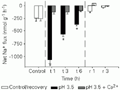
[View larger version of this image (7 K GIF file)]
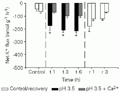
[View larger version of this image (7 K GIF file)]
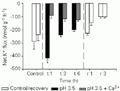
[View larger version of this image (8 K GIF file)]
Effect of calcium at pH 3.5
High [Ca2+] greatly affected ion losses in both species at pH 3.5. However, C. adolfoi was not able to control the Na+ efflux rate even in the presence of Ca2+ (Figure 1). Net Na+ flux was not significantly different in the first hour of treatment for this blackwater species (-235 ± 33 nmol g-1 h-1). During the subsequent sampling periods, Na+ loss was progressively higher, as much as 5 times and 18 times greater (-1,452 ± 176 and -4,591 ± 531 nmol g-1 h-1, respectively). These losses represent about a 70% attenuation of the losses at low [Ca2+], but were still substantially above those seen in C. schwartzi even at low [Ca2+]. For C. schwartzi, elevated [Ca2+] completely prevented the Na+ losses caused by low pH exposure. Indeed, Na+ losses were reduced by about 50% even compared to the control series at pH 6.0 (average losses were -118 ± 28 nmol g-1 h-1), indicating a positive effect of Ca2+ in the presence of acute low pH (Figure 2).
Rather different patterns were seen for K+ fluxes. C. adolfoi did not show K+ disruption when exposed to low pH under high [Ca2+] (Figure 3). The average loss in the treatment was 91 ± 10 nmol g-1 h-1, representing a net K+ flux 38% higher than the control, although not statistically different. C. schwartzi exhibited a ~50% reduction in K+ loss when exposed to low pH under high [Ca2+], accounting for an average 112 ± 16 nmol g-1 h-1 loss (Figure 4), slightly higher than that observed for C. adolfoi.
Discussion
Net Na + and K + fluxes in Corydoras
Differences in the acid tolerance were evident between the two species tested in the present study. C. schwartzi showed high acid tolerance, being very adept at dealing with rapid changes in water pH. C. adolfoi, the blackwater species, showed a marked Na+ disturbance at pH 3.5, developing massive and progressive losses. Such massive Na+ loss demonstrated for C. adolfoi does not necessarily imply that the species is acid-intolerant. The sunfish (Enneacanthus obesus), living in peat bogs at pH 3.7 in North America, also developed massive Na+ loss when submitted experimentally to pH 3.5 (14). Body Na+ levels declined by 30% after two weeks but remained stable over the following three weeks at pH 3.5. Moreover, the high Na+ disruption demonstrated for C. adolfoi is very similar to that found for other blackwater species, namely the tetra Gymnocorymbus ternetzi (15), and for the neon tetra Paracheirodon innesi (16). Like the neon tetra, C. schwartzi developed a control mechanism over subsequent periods so as to adapt to low pH. C. adolfoi did not show Na+ efflux control during the 6-h treatment period, indicating that it may take longer for C. adolfoi to adjust to the acid conditions of the transfer from pH 6.0.
It is still not clear why some predicted acid tolerant species that thrive and reproduce at extremely low pHs do not show high tolerance under experimental conditions. Blackwater fish species inhabiting the igapó areas are exposed to natural acidity due to humic and fulvic acids, that make up the dissolved organic carbon fraction. Dissolved organic carbon concentration in the flooded forest is about 35 mg/l (2). The carboxylic and phenolic hydroxyl groups of these organic acids are the main source of blackwater acidity (17). Weak acids (such as humic and fulvic types) are not completely ionized in aqueous solution, and typically have a high pKa. Conversely, inorganic acids (HNO3, H2SO4, and so forth) such as those used in the present study are almost completely ionized in solution. These conditions do not represent the same type of acidity that fish experience in the wild, even though they have the same [H+] in the water.
Given its normal circumneutral habitat in whitewater, the high tolerance of C. schwartzi under low pH conditions is quite remarkable. This species controlled both Na+ and K+ losses well at pH 3.5. In contrast to the characiform matrincha (Brycon sp) and tambaqui (Colossoma macropomum) (18), C. schwartzi seems to be much more adapted to rapid changes in water pH. The efflux rate of matrincha was reduced by 40% after 18 h, but the losses were still significant. C. schwartzi showed a 90% decrease in Na+ loss after 6 h at pH 3.5, indicating the ability to limit Na+ losses faster than matrincha.
In spite of Na+ disruption, K+ losses were under control for both species. C. schwartzi had a slightly higher K+ loss than C. adolfoi, which decreased over time for both, indicating that they were able to control the disturbance generated by low pH. Jnet K+ was closely similar to that found in a previous study for other Amazonian fish at pH 3.5 (19). It is worth mentioning that K+ efflux is an indicator of intracellular osmotic imbalance, being lost primarily from intracellular media. The fact that K+ loss remained under control may indicate that C. adolfoi was not experiencing a great intracellular ionoregulatory disturbance, which may be the key to survival.
Effects of calcium
Na+ loss in C. schwartzi was strongly influenced by [Ca2+], but this effect was less pronounced in C. adolfoi. Although the Na+ loss decreased significantly (about 70%) in relation to the pH 3.5 treatment, C. adolfoi was not able to control Na+ loss, which still increased progressively throughout exposure. Gonzalez et al. (15,19) pointed out that Ca2+ should not be involved to a greater extent in the regulation of branchial permeability at low pH. A high [Ca2+] (100-500 µmol/l) had almost no effect on ion loss in blackwater species compared to 10 µmol/l [Ca2+]. It is still unclear whether the acclimation period out of the wild conditions would induce different ionoregulatory patterns in C. adolfoi. It is reasonable to propose that Ca2+ does not play any special role in blackwater fish acid tolerance since this element is almost unavailable in the ion-poor waters of the Amazon region. This supports the idea that ion regulation in blackwater systems is modulated by different mechanisms, as previously suggested by others (15,16,18,19). Gonzalez et al. (19) suggested that humic compounds might interact directly with the branchial tight junctions and influence permeability.
In contrast, ionoregulatory control in C. schwartzi is clearly Ca2+ dependent, as shown for the tambaqui (C. macropomum), a blackwater-whitewater migratory species (12,18,20,21). This indicates that C. schwartzi has a high branchial affinity for Ca2+, particularly at the paracellular tight junctions, thus presenting diffusive ion losses.
The adaptive strategies involved in acid tolerance in the blackwaters are still a topic of discussion. The physiological adjustments that allow fish to inhabit low pH waters with low ion concentrations seem to be their ability to maintain an ionic balance by a combination of adjustments to enhance transport and limit permeability. Most reports seem to emphasize the idea that ion loss control is the key factor for species survival in acid waters, rather than maintenance of the ion uptake rates. Naturally adapted fish from soft ion-poor waters such as the Amazon blackwaters did not show branchial affinity for Ca2+, indicating modulation by other still unknown physiological mechanisms. The investigation of the effect of dissolved organic carbon compounds on ion regulation should be a fruitful field for additional research concerning the natural adaptations of fish to naturally acidic waters.
16. Gonzalez RJ & Preest MR (1999). Ionoregulatory specializations for exceptional tolerance of ion poor acidic waters in the neon tetra (Paracheirodon innesi). Physiological and Biochemical Zoology, 72: 156-163.
Acknowledgments
We thank the ornamental fish exporters in Manaus for supplying the fish, and C.M. Wood for revising the manuscript.
Address for correspondence: A.L. Val, INPA, Alameda Cosme Ferreira, 1756, 69083-000 Manaus, AM, Brasil. Fax: +55-92-643-3186. E-mail: dalval@inpa.gov.br
Research supported by CNPq and INPA. A.L. Val is the recipient of a research fellowship from CNPq, and A.Y. Matsuo was the recipient of a MSc fellowship from CAPES. Received April 11, 2001. Accepted January 21, 2002.
- 1. Furch K (1984). Water chemistry of the Amazon basin: The distribution of chemical elements among freshwaters. In: Sioli H (Editor), The Amazon. Limnology and Landscape Ecology of a Mighty Tropical River and its Basin Dr. W. Junk Publishers, Dordrecht, The Netherlands, 167-199.
- 2. Walker I (1995). Amazonian streams and small rivers. In: Tundisi JG, Matsumura-Tundisi T & Bicudo C (Editors), Limnology in Brazil Academia Brasileira de Ciências/Sociedade Brasileira de Limnologia, São Carlos, SP, Brazil, 167-193.
- 3. Val AL & Almeida-Val V (1995). Fishes of the Amazon and Their Environment. Springer-Verlag, Berlin, Germany.
- 4. McDonald DG (1983). The effects of H+ upon the gills of freshwater fish. Canadian Journal of Zoology, 61: 691-703.
- 5. Wood CM (1989). The physiological problems of fish in acid waters. In: Morris R, Taylor EW, Brown DJA & Brown JA (Editors), Acid Toxicity and Aquatic Animals. Cambridge University Press, Cambridge, UK, 125-152.
- 6. McDonald DG, Hobe H & Wood CM (1980). The influence of calcium on the physiological responses of the rainbow trout, Salmo gairdneri, to low environmental pH. Journal of Experimental Biology, 88: 109-131.
- 7. Brown DJA (1982). The effect of pH and calcium on fish and fisheries. Water, Air and Soil Pollution, 18: 343-351.
- 8. McWilliams PG (1982). The effects of calcium on sodium fluxes in the brown trout, Salmo trutta, in neutral and acid water. Journal of Experimental Biology, 96: 439-442.
- 9. Hunn JB (1985). Role of calcium in gill function in freshwater fishes. Comparative Biochemistry and Physiology, 82: 543-547.
- 10. Grizzle JM, Mauldin AC, Young D & Henderson E (1985). Survival of the juvenile striped bass (Morone saxatilis) and Morone hybrid bass (Morone chrysops x Morone saxatilis) increased by addition of calcium to soft water. Aquaculture, 46: 167-171.
- 11. Burgess WE (1987). A Complete Guide to Corydoras T.F.H. Publications, Inc., Neptune City, NJ, USA.
- 12. Wood CM, Wilson RD, Gonzalez RJ, Patrick ML, Bergman HJ, Narahara A & Val AL (1998). Responses of an Amazonian teleost, the tambaqui (Colossoma macropomum), to low pH in extremely soft water. Physiological Zoology, 71: 658-670.
- 13. Wood CM (1992). Flux measurements as indices of H+ and metal effects on freshwater fish. Aquatic Toxicology, 22: 239-264.
- 14. Gonzalez RJ & Dunson WA (1987). Adaptations of sodium balance to low pH in a sunfish (Enneacanthus obesus) from naturally acidic waters. Journal of Comparative Physiology, 157: 555-566.
- 15. Gonzalez RJ, Dalton V & Patrick ML (1998). Ion regulation in ion-poor acidic water by the blackskirt tetra (Gymnocorymbus ternetzi), a fish native to the Amazon River. Physiological Zoology, 70: 428-435.
- 17. Thurman EM (1985). Aquatic humic substances. In: Thurman EM (Editor), Organic Geochemistry of Natural Waters Martinus Nijhoff/Dr. W. Junk Publishers, Dordrecht, The Netherlands, 273-361.
- 18. Wilson RW, Wood CM, Gonzalez RJ, Patrick ML, Bergman HL, Narahara A & Val AL (1999). Ion and acid-base balance in three species of Amazonian fish during gradual acidification of extremely soft water. Physiological and Biochemical Zoology, 72: 277-285.
- 19. Gonzalez RJ, Wood CM, Wilson RW, Patrick ML, Val AL, Bergman HL & Narahara A (1998). Effects of water pH and calcium concentration on ion balance in fish of the Rio Negro, Amazon. Physiological Zoology, 71: 15-22.
- 20. Aride PHR (1998). Efeito do pH da água nos parâmetros hematológicos de Colossoma macropomum (Cuvier, 1818) mantido sob alimentação artificial. Master's thesis, INPA, Manaus, AM, Brazil.
- 21. Portela JM (1998). Efeitos do pH, cálcio e temperatura sobre a homeostase iônica de alevinos de tambaqui, Colossoma macropomum (Characiformes, Serrasalmidae). Master's thesis, INPA, Manaus, AM, Brazil.
Correspondence and Footnotes
Publication Dates
-
Publication in this collection
06 Mar 2002 -
Date of issue
Mar 2002
History
-
Received
11 Apr 2001 -
Accepted
21 Jan 2002