Abstract
Duchenne muscular dystrophy is one of the most devastating myopathies. Muscle fibers undergo necrosis and lose their ability to regenerate, and this may be related to increased interstitial fibrosis or the exhaustion of satellite cells. In this study, we used mdx mice, an animal model of Duchenne muscular dystrophy, to assess whether muscle fibers lose their ability to regenerate after repeated cycles of degeneration-regeneration and to establish the role of interstitial fibrosis or exhaustion of satellite cells in this process. Repeated degenerative-regenerative cycles were induced by the injection of bupivacaine (33 mg/kg), a myotoxic agent. Bupivacaine was injected weekly into the right tibialis anterior muscle of male, 8-week-old mdx (N = 20) and C57Bl/10 (control, N = 10) mice for 20 and 50 weeks. Three weeks after the last injection, the mice were killed and the proportion of regenerated fibers was counted and reported as a fibrosis index. Twenty weekly bupivacaine injections did not change the ability of mdx muscle to regenerate. However, after 50 weekly bupivacaine injections, there was a significant decrease in the regenerative response. There was no correlation between the inability to regenerate and the increase in interstitial fibrosis. These results show that after prolonged repeated cycles of degeneration-regeneration, mdx muscle loses its ability to regenerate because of the exhaustion of satellite cells, rather than because of an increase in interstitial fibrosis. This finding may be relevant to cell and gene therapy in the treatment of Duchenne muscular dystrophy.
mdx fiber regeneration; Bupivacaine; Duchenne muscular dystrophy; Muscle degeneration
Braz J Med Biol Res, June 2002, Volume 35(6) 691-695 (Short Communication)
Impaired regeneration of dystrophin-deficient muscle fibers is caused by exhaustion of myogenic cells
M.A.M. Luz, M.J. Marques and H. Santo Neto
Departamento de Anatomia, Instituto de Biologia, Universidade Estadual de Campinas, Campinas, SP, Brasil
Text
References
Correspondence and Footnotes Correspondence and Footnotes Correspondence and Footnotes
Abstract
Duchenne muscular dystrophy is one of the most devastating myopathies. Muscle fibers undergo necrosis and lose their ability to regenerate, and this may be related to increased interstitial fibrosis or the exhaustion of satellite cells. In this study, we used mdx mice, an animal model of Duchenne muscular dystrophy, to assess whether muscle fibers lose their ability to regenerate after repeated cycles of degeneration-regeneration and to establish the role of interstitial fibrosis or exhaustion of satellite cells in this process. Repeated degenerative-regenerative cycles were induced by the injection of bupivacaine (33 mg/kg), a myotoxic agent. Bupivacaine was injected weekly into the right tibialis anterior muscle of male, 8-week-old mdx (N = 20) and C57Bl/10 (control, N = 10) mice for 20 and 50 weeks. Three weeks after the last injection, the mice were killed and the proportion of regenerated fibers was counted and reported as a fibrosis index. Twenty weekly bupivacaine injections did not change the ability of mdx muscle to regenerate. However, after 50 weekly bupivacaine injections, there was a significant decrease in the regenerative response. There was no correlation between the inability to regenerate and the increase in interstitial fibrosis. These results show that after prolonged repeated cycles of degeneration-regeneration, mdx muscle loses its ability to regenerate because of the exhaustion of satellite cells, rather than because of an increase in interstitial fibrosis. This finding may be relevant to cell and gene therapy in the treatment of Duchenne muscular dystrophy.
Key words:mdx fiber regeneration, Bupivacaine, Duchenne muscular dystrophy, Muscle degeneration
Duchenne muscular dystrophy (DMD), caused by a defect in the dystrophin gene (1), is the most common form of muscular dystrophy. The absence of dystrophin results in muscle fiber necrosis which, in the early stages of the disease, is counterbalanced by the ability of muscle fibers to regenerate. The ability to regenerate is subsequently lost and results in progressive muscle weakness that usually confines the patient to a wheelchair by the early teens and leads to death by the early twenties (2). Understanding why muscle loses its ability to regenerate is of fundamental importance in DMD research and new insights into the mechanism of impaired muscle regeneration can be obtained by studying the mdx mouse, an animal model of DMD (3-5).
The loss of the ability to regenerate may result from the exhaustion of myogenic satellite cells caused by excessive cycles of degeneration and regeneration (6-8). However, no decline in muscle regeneration was reported in mdx mice when the muscle was subjected to repeated cycles of degeneration and regeneration (9), and there is no evidence that extensive myoblast proliferation depletes the myogenic responsiveness in later life when the animals become weak and wasted. The loss of regenerative capacity could also result from a progressive increase in muscle interstitial fibrosis since this would prevent the movement of myogenic cells, which is necessary for myotube formation (7,8). The role of fibrosis has not been well established nor is it clear why the capacity for regeneration declines in DMD and in mdx mice.
In this study, we examined whether the ability of mdx muscle to regenerate decreases after a prolonged period of repeated cycles of degeneration-regeneration, and whether this reduction was related to the exhaustion of satellite cells or to an increase in interstitial fibrosis. Our results provide a long-term in vivo analysis of the decline in the regenerative ability of muscle.
Cycles of degeneration and regeneration were induced by intramuscular injections of 5 mg/ml bupivacaine hydrochloride to provide a dose of 33 mg/kg. All experiments were done in accordance with the guidelines for the use of animals set forth by our institution. Twenty male mdx mice were anesthetized with a mixture of ketamine hydrochloride and thiazine hydrochloride (0.01 ml and 0.02 g/ml, ip) and 0.2 ml bupivacaine was injected into the upper two thirds of the right tibialis anterior muscle. Contralateral muscles were injected with 0.2 ml sterile physiological saline. The first injection was given 8 weeks after birth. Thereafter, the animals received weekly injections for 20 weeks (N = 10) and 50 weeks (N = 10). Ten C57Bl/10 mice (controls) received the same treatment.
The mice in each group were killed 3 weeks after the last injection for each period, and fixed in formol-calcium fixative for 24 h. The mice were killed with an overdose of the anesthetic after removal of the muscles. After fixation, the muscles were processed for embedding in hydroxyethyl methacrylate and 2-µm transverse sections obtained from the middle muscle belly were stained with hematoxylin and eosin.
The total cross-sectional area (TCSA), defined as the area occupied by the myofibers plus connective tissue, was measured in a single complete section of individual muscle using a 40X objective and a computer-assisted image analyzer system. The cross-sectional area of each myofiber (myofiber cross-sectional area, MCSA) was measured and the sum of all MCSA was considered to be the total myofiber cross-sectional area (TMCSA). The area of interstitial fibrosis (AIF) was then calculated by subtracting the total myofiber cross-sectional area from the total cross-sectional area (AIF = TCSA - TMCSA). The number of muscle fibers was counted on a video monitor. In the mdx mice at the age studied, as well as in the C57Bl/10 control mice treated with bupivacaine, almost all of the fibers were regenerated, which is in agreement with earlier reports for mdx (3) and after bupivacaine injections (10).
One group of 10 mdx mice received no bupivacaine or saline injections (untreated group). These mice were killed as described above at 31 and 61 weeks and the right tibialis anterior muscles were processed and examined as described for treated muscles. The data for bupivacaine-injected, saline-injected and untreated muscles from mdx mice and untreated muscles from C57Bl/10 mice were analyzed statistically using the unpaired Student t-test.
After 20 injections of bupivacaine, the muscle fiber population was not different from that observed in age-matched untreated (31 weeks old) mdx or saline-injected mdx mice. However, after 50 bupivacaine injections, the muscle fiber population decreased significantly (Table 1). This finding demonstrated that muscle regeneration depended on the number of injections and, therefore, on the number of degeneration-regeneration cycles to which muscle fibers were exposed. The observation that the regenerative capacity of mdx muscle was not affected by repeated bupivacaine injections (9) may be explained by the lower number of injections used in the latter study.
No decrease in the muscle fiber population was observed in mdx mice after 20 bupivacaine injections, despite a significant increase in the area of interstitial fibrosis, when bupivacaine-injected mdx muscles (20 injections) were compared with nontreated, age-matched mdx muscles (Table 1). The increase in the area of interstitial fibrosis concomitant with an increase in the total cross-sectional area of the muscle is well correlated to the phenomenon of pseudo-hypertrophy, classically described for DMD (2). On the other hand, the muscle fiber population decreased dramatically after 50 bupivacaine injections, but without a significant increase in the area of interstitial fibrosis when compared to muscles receiving 20 bupivacaine injections (Table 1, Figure 1). These findings demonstrate that there was no direct correlation between the increase in interstitial fibrosis and the impairment of muscle regeneration. They also suggest that the loss of regenerative ability was related to the exhaustion of myogenic cells rather than to an increase in interstitial fibrosis in mdx. The gradual increase in interstitial fibrosis as a result of repeated cycles of degeneration-regeneration would prevent the movement of myogenic cells required for myotube formation (7,8). However, our results support previous studies suggesting that an increase in interstitial fibrosis does not play a central role in the loss of regenerative ability. When mdx muscles were treated with a single injection of notexin, a potent myonecrotic agent, there was an increase in interstitial fibrosis with no change in the muscle fiber population (11). Despite the progressive fibrosis that occurs during the natural course of the disease, muscle recovery is better in young mdx mice than in a control mouse strain (12).
The observation that bupivacaine-injected mdx fibers lose their ability to regenerate, whereas bupivacaine-injected C57Bl/10 muscles do not, supports the recent suggestion that in mdx mice a lineage of myogenic cells, which is responsible for muscle repair after extreme damage, is eliminated during the course of the disease (6). We assumed that the impairment of muscle regeneration in mdx mice probably resulted from the exhaustion of this cell lineage since bupivacaine is a potent myotoxic agent and spares single cells, including satellite cells. If true, the present in vivo technique should allow us to estimate how many degenerative-regenerative cycles this subset of cells undergoes before exhaustion. We propose that after 20 repeated injections of bupivacaine, each muscle fiber presumably underwent at least 14 cycles of degeneration and regeneration, while after 50 injections, it underwent 35 cycles (13). Considering that each cycle involves four doublings of myogenic cells (14), we estimated that these cells would undergo a total of 56 doublings in the 20 bupivacaine group and 140 doublings in the 50 bupivacaine group.
The observation that interstitial fibrosis cannot account for the loss of regenerative ability has important clinical implications and may have applications in therapies for DMD. Since there is currently no cure for DMD, and since DMD cannot be eliminated by genetic counseling, cell and genetic therapies, such as myoblast transfer, have been explored as possible solutions. This approach involves the delivery of exogenous myoblasts which have to survive the normal immune rejection and migrate away between existing muscle fibers in order to form new fibers and to fuse with the host dystrophic fibers. However, experimental studies and clinical trials with this approach have met with little success (15), and there is considerable uncertainty as to whether this failure is the result of rejection of the transplanted cells or is due to the limited migration of implanted cells imposed by scar tissue. Since increased interstitial fibrosis is not related to the absence of muscle regeneration, immunological aspects should be given more consideration, including the insertion of copies of the missing gene using adeno-associated virus vectors (16,17) or the use of new immunosuppressor schedules (18), rather than trying to improve myoblast dispersion (19). This view is supported by recent findings showing that dystrophin expression in mdx mice is restored by the intravenous injection of stem cells which need to diffuse along host dystrophic and normal muscle fibers (20).
The ability of muscle to regenerate is lost in mdx mice after prolonged degenerative-regenerative cycles. This impairment is related to the exhaustion of myogenic cells rather than to an increase in scar tissue. Our results support the idea that a subset of proliferative myogenic cells that can be evoked by extreme conditions of muscle damage is impaired in mdx, but not in normal mice. These cells may have a life span greater than 56 doublings. These results may have implications for therapeutic interventions in DMD involving genetic engineering and myoblast implantation.
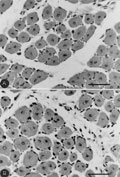
[View larger version of this image (72 K JPG file)]
Acknowledgments
The authors thank Dr. Mayana Zatz for providing the mdx mice.
Address for correspondence: H. Santo Neto, Departamento de Anatomia, Instituto de Biologia, UNICAMP, 13083-970 Campinas, SP, Brasil. Fax: +55-192-3289-3124. E-mail: marques@unicamp.br
Research supported by FAPESP (No. 95/6956-9). M.J. Marques and H. Santo Neto are supported by CNPq (Nos. 300061/99-4 and 3001053/91-0, respectively). Received November 27, 2001. Accepted March 21, 2002.
- 1. Koenig M, Monaco AP & Kunkel LM (1988). The complete sequence of dystrophin predicts a rod-shaped cytoskeletal protein. Cell, 53: 219-228.
- 2. Engel AG, Yamamoto M & Fischbeck KH (1994). Muscular dystrophies. In: Engel AG & Franzini-Armstrong C (Editors), Myology McGraw-Hill, Inc., New York, NY, USA.
- 3. Bulfield G, Siller WG, Wight PAL & Moore KJ (1984). X-chromosome linked muscular dystrophy (mdx) in the mouse. Proceedings of the National Academy of Sciences, USA, 81: 1189-1192.
- 4. Lefaucheur JP, Pastoret C & Sebille A (1995). Phenotype of dystrophinopathy in old mdx mice. Anatomical Record, 242: 70-76.
- 5. Pastoret C & Sebille A (1995). mdx mice show progressive weakness and muscle deterioration with age. Journal of Neurological Sciences, 129: 97-105.
- 6. Heslop L, Morgan JE & Partridge TA (2000). Evidence for a myogenic stem cell that is exhausted in dystrophic muscle. Journal of Cell Science, 113: 2299-2308.
- 7. Irintchev A, Zweyer M & Wernig A (1997). Impaired functional and structural recovery after muscle injury in dystrophic mdx mice. Neuromuscular Disorders, 7: 117-125.
- 8. Bockhold KJ, Rosenblatt JD & Partridge TA (1998). Aging normal and dystrophic mouse muscle: analysis of myogenicity in cultures of living single fibers. Muscle and Nerve, 21: 173-183.
- 9. Itagaki Y, Saida K & Iwamura K (1995). Regenerative capacity of mdx mouse muscles after repeated applications of myo-necrotic bupivacaine. Acta Neuropathologica, 89: 380-384.
- 10. Minatel E, Santo Neto H & Marques MJ (2001). Acetylcholine receptors and neuronal nitric oxide synthase distribution at the neuromuscular junction of regenerated muscle fibers. Muscle and Nerve, 24: 410-416.
- 11. Gross J & Morgan JE (1999). Muscle precursor cells injected into irradiated mdx mouse persist after serial injury. Muscle and Nerve, 22: 174-185.
- 12. Zacharias JM & Anderson JE (1991). Muscle regeneration after imposed injury is better in younger than older mdx dystrophic mice. Journal of Neurological Sciences, 104: 190-196.
- 13. Sadeh M, Czyzewski K & Stern LZ (1985). Chronic myopathy induced by repeated bupivacaine injections. Journal of Neurological Sciences, 67: 229-238.
- 14. Bischoff R (1994). The satellite cell and muscle regeneration. In: Engel AG & Franzini-Armstrong C (Editors), Myology McGraw-Hill, Inc., New York, NY, USA.
- 15. Smythe GM, Hodgetts SI & Grounds MD (2000). Immunobiology and the future of myoblast transfer therapy. Molecular Therapy, 1: 304-313.
- 16. Wang B, Li J & Xiao X (2000). Adeno-associated virus vector carrying human minidystrophin genes effectively ameliorates muscular dystrophy in mdx mouse model. Proceedings of the National Academy of Sciences, USA, 97: 13714-13719.
- 17. Mann CJ, Honeyman K, Cheng AJ, Ly T, Lloyd F, Fletcher S, Morgan JE, Partridge TA & Wilton SD (2001). Antisense-induced exon skipping and synthesis of dystrophin in the mdx mouse. Proceedings of the National Academy of Sciences, USA, 98: 42-47.
- 18. Camirand G, Caron NJ, Asselin I & Tremblay JP (2001). Combined immunosuppression of mycophenolate mofetil and FK506 for myoblast transplantation in mdx mice. Transplantation, 72: 38-44.
- 19. Ito H & Tremblay JP (1998). Prior culture with concanavalin A increases intramuscular migration of transplanted myoblast. Muscle and Nerve, 21: 291-297.
- 20. Gussoni E, Soheoka Y, Strickland CD, Buzney EA, Khan MK, Flint AF, Kunkel LM & Mulligan RC (1999). Dystrophin expression in the mdx mouse restored by stem cell transplantation. Nature, 401: 390-394.
Correspondence and Footnotes
Publication Dates
-
Publication in this collection
28 May 2002 -
Date of issue
June 2002
History
-
Accepted
21 Mar 2002 -
Received
27 Nov 2001