Abstract
We investigated the effects of adenosine on prolactin (PRL) secretion from rat anterior pituitaries incubated in vitro. The administration of 5-N-methylcarboxamidoadenosine (MECA), an analog agonist that preferentially activates A2 receptors, induced a dose-dependent (1 nM to 1 µM) increase in the levels of PRL released, an effect abolished by 1,3-dipropyl-7-methylxanthine, an antagonist of A2 adenosine receptors. In addition, the basal levels of PRL secretion were decreased by the blockade of cyclooxygenase or lipoxygenase pathways, with indomethacin and nordihydroguaiaretic acid (NDGA), respectively. The stimulatory effects of MECA on PRL secretion persisted even after the addition of indomethacin, but not of NDGA, to the medium. MECA was unable to stimulate PRL secretion in the presence of dopamine, the strongest inhibitor of PRL release that works by inducing a decrease in adenylyl cyclase activity. Furthermore, the addition of adenosine (10 nM) mimicked the effects of MECA on PRL secretion, an effect that persisted regardless of the presence of LiCl (5 mM). The basal secretion of PRL was significatively reduced by LiCl, and restored by the concomitant addition of both LiCl and myo-inositol. These results indicate that PRL secretion is under a multifactorial regulatory mechanism, with the participation of different enzymes, including adenylyl cyclase, inositol-1-phosphatase, cyclooxygenase, and lipoxygenase. However, the increase in PRL secretion observed in the lactotroph in response to A2 adenosine receptor activation probably was mediated by mechanisms involving regulation of adenylyl cyclase, independent of membrane phosphoinositide synthesis or cyclooxygenase activity and partially dependent on lipoxygenase arachidonic acid-derived substances.
Adenosine; Prolactin; A2 receptor; Pituitary gland
Braz J Med Biol Res, July 2002, Volume 35(7) 855-860
Stimulatory effects of adenosine on prolactin secretion in the pituitary gland of the rat
D.L.W. Picanço-Diniz1, M.M. Valença2, A.L.V. Favaretto3 and J. Antunes-Rodrigues3
1Departamento de Fisiologia, Centro de Ciências Biológicas, Universidade Federal do Pará, Belém, PA, Brasil
2Departamento de Neuropsiquiatria, Centro de Ciência da Saúde, Universidade Federal de Pernambuco, Recife, PE, Brasil
3Departamento de Fisiologia, Faculdade de Medicina de Ribeirão Preto, Universidade de São Paulo, Ribeirão Preto, SP, Brasil
References
Correspondence and Footnotes Correspondence and Footnotes Correspondence and Footnotes
Abstract
We investigated the effects of adenosine on prolactin (PRL) secretion from rat anterior pituitaries incubated in vitro. The administration of 5-N-methylcarboxamidoadenosine (MECA), an analog agonist that preferentially activates A2receptors, induced a dose-dependent (1 nM to 1 µM) increase in the levels of PRL released, an effect abolished by 1,3-dipropyl-7-methylxanthine, an antagonist of A2adenosine receptors. In addition, the basal levels of PRL secretion were decreased by the blockade of cyclooxygenase or lipoxygenase pathways, with indomethacin and nordihydroguaiaretic acid (NDGA), respectively. The stimulatory effects of MECA on PRL secretion persisted even after the addition of indomethacin, but not of NDGA, to the medium. MECA was unable to stimulate PRL secretion in the presence of dopamine, the strongest inhibitor of PRL release that works by inducing a decrease in adenylyl cyclase activity. Furthermore, the addition of adenosine (10 nM) mimicked the effects of MECA on PRL secretion, an effect that persisted regardless of the presence of LiCl (5 mM). The basal secretion of PRL was significatively reduced by LiCl, and restored by the concomitant addition of both LiCl and myo-inositol. These results indicate that PRL secretion is under a multifactorial regulatory mechanism, with the participation of different enzymes, including adenylyl cyclase, inositol-1-phosphatase, cyclooxygenase, and lipoxygenase. However, the increase in PRL secretion observed in the lactotroph in response to A2 adenosine receptor activation probably was mediated by mechanisms involving regulation of adenylyl cyclase, independent of membrane phosphoinositide synthesis or cyclooxygenase activity and partially dependent on lipoxygenase arachidonic acid-derived substances.
Key words: Adenosine, Prolactin, A2 receptor, Pituitary gland
Introduction
Adenosine is an endogenous nucleoside formed by the hydrolysis of adenosine 5'-triphosphate (ATP) that modulates many physiological processes. In addition to the well-known fundamental intracellular function exerted by ATP as the source of energy for living cells, several lines of evidence indicate that ATP is also released into the extracellular space. In this respect, the sympathetic nervous system releases the catecholamines noradrenaline and adrenaline and the purines ATP, adenosine, and inosine. Concurrent release of ATP with other transmitters has been demonstrated. These include noradrenaline, substance P (1) and acetylcholine (2). Furthermore, in some non-neuronal cells ATP release could be carrier-mediated and may involve ATP-binding cassette proteins, a ubiquitous family of transport ATPases (3).
The membrane purinergic receptors are classified into P1 or adenosine receptors and P2 receptors, primarily recognizing ATP, adenosine 5'-diphosphate (ADP), uridine 5'-diphosphate (UDP), and uridine 5'-triphosphate (UTP). Adenosine receptors have been further subdivided into four subtypes, A1, A2A, A2B, and A3, all of which couple to G proteins. The P2 receptors are divided into two families of ligand-gated ion channels and G protein-coupled receptors termed P2X and P2Y receptors, respectively. Actually, seven mammalian P2X receptors (P2X1-7) and five mammalian P2Y receptors (P2Y1, P2Y2, P2Y4, P2Y6, P2Y11) have been cloned, characterized, and accepted as valid members of the P2 receptor family (4).
We have shown that dipyridamol, a blocker of adenosine transport, did not modify prolactin (PRL) secretion from hemipituitaries incubated in vitro, although it enhanced PRL stimulation induced by adenosine (5). These data demonstrated that a purinergic effect occurred mainly due to outer membrane receptors and that no effects of endogenous adenosine were observed in this type of in vitro preparation. Indeed, adenohypophyseal cell lines release adenosine together with the enzyme adenosine deaminase (6), suggesting that adenosine may act through an autocrine mechanism and that its levels may be regulated within strict limits.
Finally, adenosine analogues may have opposite effects on PRL secretion by modulating adenylyl cyclase activity (7), resulting in either an increase or a decrease in cyclic AMP levels in the lactotroph (8). These effects may be related to the affinity of the receptor for different concentrations or structural groups of the agonist.
In the present study we investigated the activation of A2 receptors in the regulation of PRL secretion by the rat anterior pituitary gland in vitro. Interactions with dopamine as well as the involvement with adenylyl cyclase, inositol-1-phosphatase, cyclooxygenase, and lipoxygenase were investigated during purinergic stimulation of PRL secretion.
Material and Methods
Male Wistar rats weighing 200-220 g from the central Animal House of the Faculty of Medicine of Ribeirão Preto, University of São Paulo, were used. The animals were kept in collective cages in an artificially controlled environment with temperature ranging from 22 to 24ºC on a 12-h light-dark cycle, with free access to solid food and water.
Drugs and solutions
The following drugs were used: 5-N-methylcarboxamidoadenosine (MECA) and 1,3-dipropyl-7-methylxanthine (DPMX) (Research Biochemicals Incorporated, Natick, MA, USA); nordihydroguaiaretic acid (NDGA), indomethacin, bovine albumin, Earle salt solution and HEPES (Sigma, St. Louis, MO, USA); LiCl and ascorbic acid (Merck S.A. Indústrias Químicas, Rio de Janeiro, RJ, Brazil).
Nutrient solution consisted of Earle salt solution with 0.1% bovine albumin and 15 mM HEPES added, pH 7.4. Dopamine (10 µM) was added with ascorbic acid (100 µM) to the nutrient solution to prevent degradation.
Experimental procedures
After a period of adaptation of approximately 60 min to the laboratory, the animals were sacrificed by decapitation at 10:00 am for all experiments. The brain was removed and the anterior pituitary dissected in situ. The anterior pituitary was divided into two approximately equal parts and immersed in refrigerated nutrient solution (4ºC). Each hemipituitary was transferred to individual cuvettes containing 1.0 ml nutrient solution (37ºC) and preincubated for 60 min in a Dubnoff water bath with constant shaking for washing and to stabilize basal hormonal secretion. The specific incubations were held immediately after a change of the nutrient solution used for preincubation. After incubation the samples were placed in plastic tubes at -20ºC and the hemipituitaries were weighed on a torsion scale. The values concerning the concentrations of PRL release into the nutrient solution were divided by the wet weight (mg) of the hemipituitaries and reported as ng/mg tissue. At the end of each experiment, 56 mM KCl was added to evaluate the functional viability of cells on the basis of PRL release from intracellular stores. A considerable increase (P<0.001) in basal PRL secretion (337 ± 26 ng/mg tissue) was induced by the addition of 56 mM KCl (1,362 ± 86 ng/mg tissue), indicating that the cells maintained their secretory response for more than 135 min of incubation, thus guaranteeing the viability of the tissue preparation. The basal value (control group) was obtained from hemipituitaries incubated with fresh medium.
Radioimmunoassay
PRL concentration in the nutrient solution was determined by double-antibody radioimmunoassay (RIA). The hormones for radioiodination and specific antibodies were obtained from the National Institute of Arthritis, Diabetes and Digestive Diseases (NADDK, Baltimore, MD, USA) Rat Pituitary Hormone Program.
Statistical analysis
Data are reported as means ± SEM. Statistical analysis was performed by analysis of variance (ANOVA) for all samples and by the unpaired Student t-test for comparison between groups, with the level of significance set at P<0.05.
Results
Effects of A2 receptor activation by MECA on basal PRL secretion
The A2adenosine receptor agonist MECA at the doses of 1 to 1,000 nM induced a stimulatory dose-dependent effect on PRL secretion. The three-fold increase in PRL release reached a peak with 1,000 nM MECA. The stimulatory potency of these effects of MECA were reduced at the dose of 10,000 nM (Figure 1).
Different doses of DPMX (10-1,000 nM), a selective adenosine A2antagonist, had no effect on basal PRL secretion. The highest dose of DPMX used (1,000 nM) caused total blockade of the effect of MECA on PRL release (Figure 2).
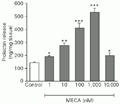
[View larger version of this image (7 K GIF file)]
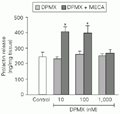
[View larger version of this image (8 K GIF file)]
Effects of MECA administration on PRL secretion inhibited by dopamine
Administration of 10 µM dopamine induced a significant decrease in the amount of PRL released into the incubation medium. None of the different MECA doses (1-1,000 nM) administered after 15 min of preincubation with 10 µM dopamine modified the PRL inhibition induced by dopamine (Figure 3).
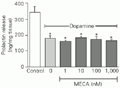
[View larger version of this image (6 K GIF file)]
Effects of adenosine administration on the secretion of PRL inhibited by LiCl
In order to determine whether membrane phosphoinositide synthesis interfered with basal or adenosine-induced PRL secretion, 5 mM LiCl (a blocker of inositol-1-phosphatase) was added alone or in combination with 10 nM adenosine to the nutrient solution. LiCl induced a significant decrease in basal PRL secretion. Adenosine caused a two-fold increase in basal PRL secretion, an effect not modified by combination with LiCl (Figure 4). In turn, 1 mM myo-inositol did not change basal PRL secretion. However, myo-inositol stimulated PRL release in the presence of LiCl, bringing it back to the normal control level.
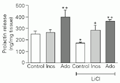
Effect of the administration of 5 mM LiCl or 10 nM adenosine (Ado) and their combination on prolactin secretion. Addition of 1 mM myo-inositol (Inos) alone or in combination with 5 mM LiCl was performed to test specificity of LiCl blocking effects. Data are reported as means ± SEM (N = 5). *P<0.05 compared to control,
[View larger version of this image (6 K GIF file)]
Effects of MECA administration on the secretion of PRL inhibited by indomethacin and NDGA
In this experiment we used indomethacin and NDGA, which inhibit cyclooxygenase and lipoxygenase activities, respectively. Both, 10 µM indomethacin and 10 µM NDGA induced a significant reduction in basal PRL levels (Figure 5). MECA (10 nM) induced an increase in PRL release both under basal conditions and in the presence of indomethacin, being ineffective in the presence of NDGA (Figure 6).
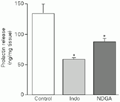
[View larger version of this image (5 K GIF file)]
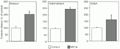
[View larger version of this image (18 K GIF file)]
Discussion
Activation of A2 purinoceptors induced PRL release from hemipituitaries incubated in vitro, an effect inhibited by lipoxygenase but not cyclooxygenase blockade. Inhibition of the enzyme inositol-1-phosphatase also did not alter the stimulatory effect of adenosine on PRL secretion. In addition, the inhibitory effect of dopamine on PRL release was not counteracted by A2 receptor activation. This suggests that the A2 receptor mainly activates adenylyl cyclase, with some dependence on lipoxygenase activity.
These data are supported by the fact that adenosine analogues had stimulatory or inhibitory effects on adenylyl cyclase activity in preparations of adenohypophyseal cells in culture (7). In fact, in these preparations MECA induced a dose-dependent increase in adenylyl cyclase activity, confirming preferential activation of A2 receptor. If we extrapolate this information to our results, we infer that both A1 and A2 receptors occur in the lactotroph since the biphasic effect on PRL secretion was observed at MECA doses of 1 to 10 µM. Recent studies have demonstrated an increase in PRL after administration of an A1 agonist (9) or A2A agonist and a decrease in PRL secretion after administration of an A1 agonist in vitro (10). These apparently contradictory results suggest that the differences may depend on the presence or absence of adenosine in the preparation and/or the type of agonist administered. If we consider the possibility that the activation of dopamine receptors (D2) in the adenohypophysis modulated membrane PGi (11,12) and inhibited adenylyl cyclase (13), we can infer that the activation of A2 receptors induced by MECA depends on the availability of PGs, which may be blocked by D2 activation.
Independently of purinergic PRL control, eicosanoids seem to be involved in the regulatory mechanisms of PRL secretion, since the inhibition of PG and thromboxane synthesis by indomethacin and of leukotrienes and hydroxyeicosatetraenoic acid by NDGA resulted in a reduction in basal PRL release. These data confirm similar effects obtained by other investigators (14,15).
Lithium (administered as LiCl) inhibits the hydrolysis of L- and B-myo-inositol-1-phosphate by the enzyme inositol-1-phosphatase, reducing the supply of myo-inositol for the cycle of membrane phosphoinositide synthesis in the cell (16). The administration of 5 mM LiCl inhibited basal PRL secretion. Its addition in combination with adenosine did not prevent the increase in PRL secretion induced by the nucleoside, suggesting that this effect does not depend on the intracellular levels of membrane phosphoinositides.
Another question is: What is the origin of adenosine at the pituitary interstitial level? We can postulate four different sources: a) from the systemic circulation, b) locally released, c) from nerve terminals in the median eminence into the portal-hypophyseal blood, and d) from direct innervation of the sympathetic nervous system of brain origin or partly originating in the superior cervical ganglia (17).
The superior cervical ganglia provide sympathetic innervation to several cephalic regions, such as pineal gland, blood vessels (including hypothalamic and pituitary gland), choroid plexus, carotid body, and salivary and thyroid glands. Cardinali et al. (18) proposed that the superior cervical ganglia can function as a peripheral neuroendocrine center. Tan and Ogawa (19) demonstrated that the sympathetic nervous system can play an important role in maintaining and regulating the secretory function of the adenohypophysis since superior cervical sympathetic ganglionectomy modified the hormonal secretory pattern.
Finally, these results provide suggestive evidence for a stimulatory action of adenosine on PRL secretion in a putative mechanism mediated by A2 receptors, partially dependent on cAMP synthesis and leukotrienes and possibly dissociated from the synthesis of membrane phosphoinositides and PG or thromboxanes.
Acknowledgments
We thank Rubens Fernando de Melo, Leonardo Fidelis Filho, Gilberto Lopes, Manoel Corrêa de Lima, Marina Holanda, and Maria Valci Aparecida dos Santos Silva for technical assistance.
Address for correspondence: J. Antunes-Rodrigues, Departamento de Fisiologia, FMRP, USP, Av. Bandeirantes, 3900, 14049-900 Ribeirão Preto, SP, Brasil. E-mail: antunes@fmrp.usp.br
Research supported by FAPESP (Nos. 91/0567-0 and 94/3805-7) and CNPq (Nos. 50167/91-7 and 521593/94-8). Received August 29, 2001. Accepted May 7, 2002.
- 1. Matsuka Y, Neubert JK, Maidment NT & Spigelman I (2001). Concurrent release of ATP and substance P within guinea pig trigeminal ganglia in vivo Brain Research, 915: 248-255.
- 2. Schweitzer E (1987). Coordinated release of ATP and ACh from cholinergic synaptosomes and its inhibition by calmodulin antagonists. Journal of Neuroscience, 7: 2948-2956.
- 3. Bodin P & Burnstock G (2001). Purinergic signalling: ATP release. Neurochemical Research, 26: 59-69.
- 4. Ralevic V & Burnstock G (1998). Receptors for purines and pyrimidines. Pharmacological Reviews, 50: 413-492.
- 5. Picanço-Diniz DLW, Valença MM, Favaretto ALV & Antunes-Rodrigues J (1992). Dipyridamole amplifies the effects of adenosine on gonadotropin and prolactin release from the rat anterior pituitary gland. Medical Science Research, 20: 783-785.
- 6. Dorflinger LJ & Schonbrunn A (1985). Adenosine inhibits prolactin and growth hormone secretion in a clonal pituitary cell line. Endocrinology, 117: 2330-2338.
- 7. Anand-Srivastava MB, Gutkowska J & Cantin M (1985). Adenosine-sensitive adenylate cyclase in rat anterior pituitary. Neuroendocrinology, 41: 113-118.
- 8. Delahunty TM, Cronin MJ & Linden J (1988). Regulation of GH3-cell function via adenosine A1 receptors. Inhibition of prolactin release, cyclic AMP production and inositol phosphate generation. Biochemical Journal, 255: 69-77.
- 9. Yu WH, Kimura M, Walczewska A, Porter JC & McCann SM (1998). Adenosine acts by A1 receptors to stimulate release of prolactin from anterior pituitaries in vitro Proceedings of the National Academy of Sciences, USA, 95: 7795-7798.
- 10. Kumari M, Buckingham JC, Poyser RH & Cover PO (1999). Roles for adenosine A1- and A2-receptors in the control of thyrotrophin and prolactin release from the anterior pituitary gland. Regulatory Peptides, 79: 41-46.
- 11. Senogles SE, Benovic JL, Amlayk N, Unson C, Milligan G, Vinitsky R, Spielgel M & Caron MG (1987). The D2 dopamine receptor of anterior pituitary is functionally associated with a pertussis toxin-sensitive guanine nucleotide. Journal of Biological Chemistry, 262: 4860-4867.
- 12. Burris TP, Nguyen DN, Smith SG & Freeman ME (1992). The stimulatory and inhibitory effects of dopamine on prolactin secretion involve different G-proteins. Endocrinology, 130: 926-932.
- 13. Enjalbert A & Bockaert J (1983). Pharmacological characterization of D2 dopamine receptor negatively coupled with adenylate cyclase in rat anterior pituitary. Molecular Pharmacology, 23: 576-584.
- 14. McCann SM, Rettori V, Milenkovic L, Jurcovicova J & Gonzalez MC (1990). Role of monokines in control of anterior pituitary hormone release. Advances in Experimental Medicine and Biology, 274: 315-329.
- 15. Miyake A, Nishizaki T, Ikegami H, Koike K, Hirota K & Tanizawa O (1988). Possible involvement of lipoxygenase pathway of arachidonic acid in rat pituitary hormone release in vitro Journal of Endocrinological Investigation, 11 (Suppl 11): 805-808.
- 16. Hallcher LM & Sherman WR (1980). The effects of lithium ion and other agents on the activity of myo-inositol-1-phosphatase from bovine brain. Journal of Biological Chemistry, 25: 10896-10901.
- 17. Saavedra JM (1985). Central and peripheral catecholamine innervation of the rat intermediate and posterior pituitary lobes. Neuroendocrinology, 40: 281-284.
- 18. Cardinali DP, Vacas MI, Gejman PV, Pisarev MA, Barontini M, Boado RJ & Juvenal GJ (1993). The sympathetic superior cervical ganglia as "little neuroendocrine brains". Acta Physiologica, Pharmacologica et Therapeutica Latinoamericana, 33: 205-221.
- 19. Tan Y & Ogawa H (1996). Effect of superior cervical sympathetic ganglionectomy on FSH, LH and GH cells of hypophyseal gland in female rats: a quantitative immunohistochemical study. Masui, 45 (Suppl 10): 1223-1234.
Correspondence and Footnotes
Publication Dates
-
Publication in this collection
16 July 2002 -
Date of issue
July 2002
History
-
Accepted
07 May 2002 -
Received
29 Aug 2001