Abstract
Based on kinetic theory of gases and on the combined method of Chapman-Enskog and Grad, the laws of Ohm, Fourier and Navier-Stokes are derived for a non-relativistic fully ionized gas. Moreover, the combined method is applied to the BGK model of the relativistic Boltzmann equation and the Ohm's law is derived for a relativistic fully ionized gas.
Transport Processes in Ionized Gases
G. M. Kremer
Departamento de Física, Universidade Federal do Paraná
Caixa Postal 19081, 81531-990, Curitiba, SP, Brazil
Received October 1, 1996
Based on kinetic theory of gases and on the combined method of Chapman-Enskog and Grad, the laws of Ohm, Fourier and Navier-Stokes are derived for a non-relativistic fully ionized gas. Moreover, the combined method is applied to the BGK model of the relativistic Boltzmann equation and the Ohm's law is derived for a relativistic fully ionized gas.
I. Non-relativistic ionized gases
We may say that the objective of thermodynamics of a non-relativistic ionized gas is the determination of the five fields of mass density , velocity vi and temperature T in all points of the fluid and at all times. To achieve this objective, we need five field equations that are based on the balance equations of mass density
, momentum density
and internal energy density
, which read
In the above equations Ii is the electric current density, pij the pressure tensor and qi the heat flux vector. Moreover, E*i = Ei + (v x B)i with E denoting the external electric field, B the external magnetic flux density and s the electrical charge density. The system of balance equations (1) is closed by considering Ii , pij , e and qi as constitutive quantities that are related to the values of the basic fields in a materially dependent manner through constitutives relations.
Here we are interested in a fully ideal ionized gas and we shall base on the kinetic theory of gases to determine the constitutive equations for and qi , since in this case e and
are known functions of (
,T) . In kinetic theory of gases the state of a fully non-relativistic ionized gas is characterized by the set of one-particle distribution functions fa(x, c, t) where a = E,I denotes the constituents of a mixture of electrons and ions. The one-particle distribution function is such that f (x, ca, t)d 3xd 3ca gives at time t , the number of a particles in the volume element d 3xd 3ca around the particle position x and velocity ca. The one-particle distribution function of constituent a obeys the Boltzmann equation:
.(2)
In equation (2) ea and ma denote respectively the electric charge and the mass of a particle of constituent a , g ba = c b - c a is the relative veloctiy of two particles before collision, b and e are impact parameter, and the primes refer to after collision velocities, with f 'b denoting f (x ,c'b,t) and so forth.
We characterize a macroscopic state of the ionized gas by the fields of vi velocity of the mixture,
a partial mass density,
(3)
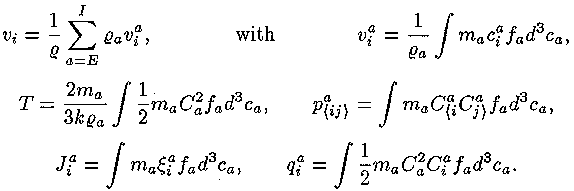
In the above equations is the mass density of the mixture, via the velocity of constituent a , k the Boltzmann constant, Cia = cia - via and
partial peculiar velocities, and we have supposed that the constituents in the mixture are at the same temperature T .
The Grad distribution function [1] corresponding to the fields defined above is:
(7)
where
is the Maxwellian distribution function.
By applying the method of Chapman-Enskog [2] for the Grad distribution function (which is the scheme of the combined method of Chapman-Enskog and Grad [3]), it follows an equation that relates the fluxes (partial pressure deviator, partial diffusion flux, partial heat flux vector) with the forces (gradient of velocity, gradient of temperature and external electric field) that reads
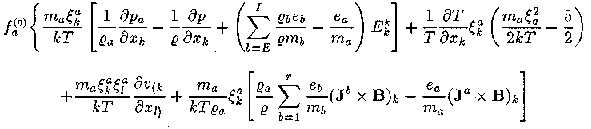

where
(9)
with
. (10)
If we multiply equation (8) successively by and integrate the resulting equations over all values of xa , we get a system of linear equations for
, Jia, qia, which can be solved by inverting second- and fourth-order tensors. The constitutive equations for the total heat flux vector qi , for the total electric current Ii and for the pressure deviator
of the mixture in a linearized theory follow from:
(11)
They read
(12)
(13)
where is a combined electric field with ma denoting the chemical potential of constituent a . Equation (12) 1 represents the law of Fourier, while (12) 2 the law of Ohm. In these equations kij is the thermal conductivity tensor, sij is the electric conductivity tensor, while Qij ,
are coefficients of cross effects. Equation (13) is the mathematical expression of the Navier-Stokes law, with
denoting the four-order tensor of shear viscosity. The general expression for the second-order tensors in terms of the magnetic flux density is:
(14)
while that for the four-order tensor reads:
(15)
In equations (14) and (15) a1 through b5 are scalar coefficients, the expressions for these coefficients in terms of the collision integrals of the Boltzmann equation are given in [3]. Moreover, the following Onsager reciprocity relations hold for the coefficients:
(16)
.(17)
II. Relativistic ionized gases
The objective of thermodynamics of relativistic ionized gases is the determination of the five fields of particle four-flow and temperature T in all events
. To determine the five fields, we refer to the following balance equations for the particle four-flow
and for the energy-momentum tensor
:
(18)
where is the electromagnetic field tensor and
the charge four-vector.
Using the four-velocity and the projector
where
is the metric tensor with signature (1,-1,-1,-1), we introduce the following decompositions
(19)
.(20)
where:
(21)
To close the system of balance equations (18) we have to consider ,
,
and
as constitutive quantities that are related to the basic fields through constitutive equations, since for ideal gases p and e are known functions of (n,T) .
We shall base on the kinetic theory of gases to get the constitutive equations. The state of a relativistic fully ionized gas in kinetic theory is characterized by the set of one-particle distribution functions (a = E,I) such that
, gives at time t , the number of a particles in the volume element d 3x about
and momenta in a range d 3pa around
. Here we are interested in a simple derivation of Ohm's law, and for that purpose we write down the BGK model [4] of the Boltzmann equation for the electrons, which reads:
(22)
where is a relaxation time,
denotes the momentum four-vector for the electrons and
(23)
is the Maxwell-Jüttner distribution function, with denoting the modified Bessel function.
According to the kinetic theory of gases (see for example [5]), the electric current is defined in terms of the diffusion flux
of constituent a by:
(24)
The Grad distribution function for the constituent a by considering only the diffusion flux is given by:
where .
Now by applying the combined method of Chapman-Enskog and Grad it follows
(26)
where hE is the enthalpy per particle of the electrons.
If we multiply the above equation by , integrate the resulting equation over all values of d 3pE/p 0E and eliminate the time derivative of the four-velocity by the use of the balance of linear momentum of the mixture for a gas where
(27)
we get
(28)
In the above equations we have introduced the time derivative
(29)
By solving equation (28) for the current four-vector, we get the mathematical expression of the relativistic Ohm's law
(30)
where the electric conductivity tensor is given by
.(31)
In equation (31) the coefficients a , b and d read
, (32)
(33)
Acknowledgements
The author gratefully acknowledges the support of the Conselho Nacional de Desenvolvimento Científico e Tecnológico of Brazil.
- 1. Grad, H., On the Kinetic Theory of Rarefied Gases, Commun. Pure Appl. Math., 2 (1949), 331-407.
- 2. Chapman, S., Cowling, T. G., The Mathematical Theory of Nonuniform Gases, Cambridge University Press, Cambridge, 1970.
- 3. Rodbard, M. G., Bezerra Jr., A. G., Kremer, G. M., A Combined Chapman-Enskog and Grad Method. II. Ionized Gases, Phys. Plasmas 2 (1995), 642-648.
- 4. Bhatnagar, P. L., Gross, E. P., Krook, M., A Model for Collision Processes in Charged and Neutral One-Component Systems, Phys. Rev. 94 (1954), 511-525.
- 5. de Groot, S. R., van Leeuwen, W. A., van Weert, Ch. G., Relativistic Kinetic Theory, North-Holland, Amsterdam, 1980.
Publication Dates
-
Publication in this collection
04 Feb 1999 -
Date of issue
Sept 1997
History
-
Received
01 Oct 1996