Abstract
We discuss the history of CP violation and its manifestations in kaon physics, its explanation in terms of phases of the Cabibbo-Kobayashi-Maskawa matrix describing charge-changing weak quark transitions, predictions for experiments involving B mesons, and the light it can shed on physics beyond the Standard Model.
CP Violation: Past, Present, and Future
Jonathan L. Rosner
Enrico Fermi Institute and Department of Physics
University of Chicago, Chicago, IL 60637 USA
Received on 3 January, 2001
We discuss the history of CP violation and its manifestations in kaon physics, its explanation in terms of phases of the Cabibbo-Kobayashi-Maskawa matrix describing charge-changing weak quark transitions, predictions for experiments involving B mesons, and the light it can shed on physics beyond the Standard Model.
I Introduction
CP symmetry and its violation are important guides to fundamental quark properties and to the understanding of the matter-antimatter asymmetry of the Universe. In this review, an updated version of one presented earlier in the year [1], we describe past, present, and future aspects of CP violation studies. After an illustration of fundamental iscrete symmetries in Maxwell's equations (Sec. II), we recall the history of CP violation's discovery [2] in the decays of neutral kaons (Sec. III). The product CPT so far seems to be conserved, as is expected in local Lorentz-invariant quantum field theories [3]. We then discuss the electroweak theory's explanation of CP violation [4] in terms of phases of the Cabibbo-Kobayashi-Maskawa (CKM) [4, 5] matrix in Sec. IV), and mention some present tests of this theory with kaons (Sec. V) B mesons (Sec. VI), and charmed particles (Sec. VII). The future of CP violation studies (Sec. VIII) is very rich, with a wide variety of experiments relevant to physics beyond the Standard Model and the baryon asymmetry of the Universe.
II Discrete symmetries in Maxwell's equations
The behavior of the Maxwell equations under the discrete symmetries P (parity), T (time reversal), C (charge conjugation), and CPT is summarized in Table I. Each term behaves as shown.
Under P, we have Electric fields change in sign while magnetic fields do not, and currents change in direction. Under T,
Magnetic fields change in sign while electric fields do not, since directions of currents are reversed. Under C,
Both electric and magnetic fields change sign, since their sources r and j change sign. Finally, under CPT, space and time are inverted but electric and magnetic fields retain their signs:
A fundamental term in the Lagrangian behaving as E · B, while Lorentz covariant, would violate P and T. Such a term seems to be strongly suppressed, in view of the small value of the neutron electric dipole moment. Its absence is a mystery, but several possible reasons have been proposed (see, e.g., [6]).
III CP symmetry for kaons
Some neutral particles, such as the photon, the neutral pion, and the Z0, are their own antiparticles, while some - those carrying nonzero quantum numbers - are not. The neutral kaon K0, discovered in 1946 [7] in cosmic radiation, was assigned a "strangeness" quantum number S = 1 in the classification scheme of Gell-Mann and Nishijima [8] in order to explain its strong production and weak decay. Production would conserve strangeness, while the weaker decay process would not. For this scheme to make sense it was then necessary that there also exist an anti-kaon, the 0, with S = -1.
As Gell-Mann described this scheme at a seminar at the University of Chicago, Enrico Fermi asked him what distinguished the
0 from the K0 if both could decay to pp, as seemed to be observed. This question led Gell-Mann and Pais [9] to propose that the states of definite mass and lifetime were
with the K1 allowed by C invariance (then thought to be a property of weak interactions) to decay to pp and the K2 forbidden to decay to pp. The K2 would be allowed to decay only to three-body final states such as p+p- p0 and thus would have a much longer lifetime. It was looked for and found in 1956 [10]. The discovery that the weak interactions violated C and P but apparently preserved the product CP [11] led to a recasting of the above argument through the identification CP(K1) = +(K1), CP(K2) = -(K2).
The K1-K2 system can be illustrated using a degenerate two-state example such as a pair of coupled pendula [12] or the first excitations of a drum head. There is no way to distinguish between the basis states illustrated in Fig. 1(a), in which the nodal lines are at angles of ±45° with respect to the horizontal, and those in Fig. 1(b), in which they are horizontal and vertical.
Basis states for first excitations of a drum head. (a) Nodal lines at ±45° with respect to horizontal; (b) horizontal and vertical nodal lines.
If a fly lands on the drum-head at the point marked "×", the basis (b) corresponds to eigenstates. One of the modes couples to the fly; the other doesn't. The basis in (a) is like that of (K0, 0), while that in (b) is like that of (K1, K2). Neutral kaons are produced as in (a), while they decay as in (b), with the fly analogous to the pp state. The short-lived state (K1, in this CP-conserving approximation) has a lifetime of 0.089 ns, while the long-lived state (
K2) lives ~ 600 times as long, for 52 ns.
In 1964 Christenson, Cronin, Fitch, and Turlay [2] found that indeed one in about 500 long-lived neutral kaons did decay to p+p-, and one in about 1000 decayed to p0p0. The states of definite mass and lifetime could then be written, approximately, as
with a parameter whose magnitude was about 2 × 10-3 and whose phase was about 45°. Since the states of definite mass and lifetime were no longer CP eigenstates, CP had to be violated somewhere. However, for many years
was the only parameter describing CP violation. One could measure its magnitude and phase more and more precisely (including learning about Re(
) through a study of charge asymmetries in KL ® p±l
n), but its origin remained a mystery. One viable theory included a "superweak" one [13] which postulated a new interaction mixing K0 = d
and
0 = s
but with no other consequences.
Kobayashi and Maskawa offered a new opportunity to describe CP violation by boldly postulating three quark families [4] when charm (the last member of the second family) had not yet even been firmly established. In the diagram of Fig. 2 describing the second-order weak transition d® s
through intermediate states involving pairs of quarks i, j = u, c, t with charges 2/3, the phases of complex weak couplings can have physical effects. As long as there are at least three quark families, one cannot redefine quark phases so that all such couplings are real, and one can generate a nonzero value of
.
The time-dependence of the two-component K0 and 0 system is governed by a 2 × 2 mass matrix
[14]:
where = M - i G/2, and M and G are Hermitian matrices. The eigenstates (11) then correspond to the eigenvalues mS,L = mS,L - i gS,L/2, with
Using data and the magnitude of CKM matrix elements one can show [14] that the second term dominates. Since the mass difference mL - mS and width difference gS- gL are nearly equal, the phase of mL- mS is about p/4, so that the phase of is also p/4 (mod p).
It is easy to model the CP-conserving neutral kaon system in table-top systems with two degenerate states [12]. The demonstration of CP violation requires systems that emulate Im(M12) ¹ 0 or Im(G12) ¹ 0. One can couple two identical resonant circuits "directionally" to each other (see Fig. 3 so that the energy fed from circuit 1 to circuit 2 differs from that fed in the reverse direction [15]. Devices with this property utilize Faraday rotation of the plane of polarization of radio-frequency waves; some references may be found in [16]. This asymmetric coupling also is inherent in the equations of motion of a spherical (or "conical") pendulum in a rotating coordinate system [17], so that the Foucault pendulum is a demonstration (though perhaps not "table-top") of CP violation. A ball rolling with viscous damping in a rotating vase of elliptical cross section holds more promise for a laboratory setting [16]. In all such cases the CP-violating effect is imposed "from the outside," leaving open the question of whether some "new physics" is governing the corresponding effect in particle physics.
IV Kobayashi-Maskawa theory of CP violation
The interactions of quarks with W± bosons are described by
where the primed quarks are "weak eigenstates":
In the weak-eigenstate basis, the mass term in the Lagrangian,
will involve a general 3 × 3 matrix , which requires separate left and right unitary transformations
to obtain a diagonal matrix LQ with non-negative entries. If we define unprimed (mass) eigenstates by
the interaction Lagrangian may be expressed as
where V º LD is the Cabibbo-Kobayashi-Maskawa (CKM) matrix. As a result of its unitarity, VV = VV = 1, the Z
couplings in the electroweak theory are flavor-diagonal. Since it contains no information about RU or RD, V provides only partial information about
Q.
For n u-type quarks and n d-type quarks, V is n × n. Since it is unitary, it can be described by n real parameters. Relative quark phases account for 2n-1 of these, leaving n2- (2n-1) = (n-1)2 physical parameters. Of these, n (n - 1)/2 (the number of independent rotations in n dimensions) correspond to angles, while the rest, (n-1)(n-2)/2, correspond to phases.
For n = 2, we have one angle and no phases. The matrix V then can always be chosen as orthogonal [5, 18]. For n = 3, we have three angles and one phase, which in general cannot be eliminated by arbitrary choices of phases in the quark fields. It was this phase that motivated Kobayashi and Maskawa [4] to introduce a third quark doublet in 1973 when only two were known. (The bottom quark was discovered in 1977 [19], and the top in 1994 [20].) The Kobayashi-Maskawa theory provides a potential source of CP violation, serving as the leading contender for the observed CP-violating effects in the kaon system and suggesting substantial CP asymmetries in the decays of mesons containing b quarks. The pattern of charge-changing weak transitions among quarks is depicted in Fig. 4.
Pattern of charge-changing weak transitions among quarks. Solid lines: relative strength 1; dashed lines: relative strength 0.22; dot-dashed lines: relative strength 0.04; dotted lines: relative strength £ 0.01. Breadths of levels denote estimated errors in quark masses.
A convenient parametrization of the CKM matrix utilizes a hierarchy [21] whereby magnitudes of elements are approximately powers of l º sinqc 0.22, where qc is the Gell-Mann-Lévy-Cabibbo angle [5, 22] describing strange particle decays. The matrix may be expressed as
where rows denote u, c, t and columns denote d, s, b.
We learn |Vcb| = A l2 0.041 ± 0.003 from the dominant decays of b quarks, which are to charmed quarks [23, 24]. Smaller errors are quoted in most reviews [25] which take different views of the dominantly theoretical sources of error. As an indication that this number is still in some flux we note a new measurement |Vcb| = 0.046 ± 0.004 by the CLEO group [26].) Similarly, we shall take from charmess b decays |Vub/Vcb| = 0.090 ± 0.025 = l(r2 + h2)1/2 [27], leading to r2 + h2 = 0.41 ± 0.11, whereas smaller errors are quoted by most authors.
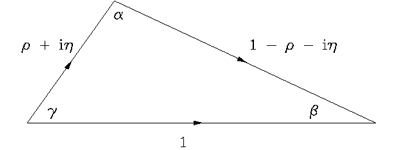

As a result of the unitarity of the CKM matrix, the quantities /Al3 = r+ ih, Vtd/Al3 = 1 - r - ih, and 1 form a triangle in the (r, h) plane (Fig. 5). We still do not have satisfactory limits on the angle g of this "unitarity triangle." Further information comes from the following constraints:
1. Mixing of neutral B mesons is dominated by top quark contributions to graphs such as Fig. 2 but with external quarks d for B0 or s
for Bs. For example, the mass splitting in the nonstrange neutral B system is
where fB is the B meson decay constant and BB = (1) is the "vacuum saturation factor," describing the degree to which graphs such as Fig. 2 describe the mixing. Recent estimates [28] give fB
= 230 ± 40 MeV. Consequently, one finds [23] |1 - r - ih| = 0.87 ± 0.21. Neutral strange B mesons are characterized by [29]
Since |Vts| |Vcb| is approximately known, this information mainly serves to constrain the product fBs
and, given information on the ratio of strange and nonstrange constants [30], the value of |Vtd|, leading to |1-r-ih| < 1.01. The large top mass, mt = 174 ± 5 GeV [31], is crucial for these mixings to be so large.
2. CP-violating K0-0 mixing through the box graphs of Fig. 2 accounts for the parameter [31]
leading to a constraint [23, 24]
Here we have used fK = 161 MeV and BK = 0.87 ± 0.13 [32]. If top quarks were fully dominant the left-hand side of this equation would be just h(1-r). The term 0.39 in brackets is a correction due to charmed quarks.
Region of (r, h) specified by constraints on CKM matrix parameters. Solid semicircles denote limits based on |Vub/Vcb| = 0.090 ± 0.025; dashed arcs denote limits 0.66 £ |1 - r- i h| £ 1.08 based on B0-
The constraints are plotted on the (r, h) plane in Fig. 6. Also shown are the ±1s bounds on sin 2b, to be discussed presently, from an average 0.49 ± 0.23 [24] of OPAL, ALEPH, CDF, BaBar, and BELLE values. The allowed region is larger than that favored by many other analyses [25].
V The CKM matrix and predictions for kaon physics
V.1 KS,L ® pp rates
If we define
the possibility of different CP-violating effects in pp states of isospin Ipp =2 and Ipp = 0 [33] gives rise to a parameter ¢ such that h+- =
+
¢, h00 =
- 2
¢. The following ratio of ratios then can differ from unity:
The ratio ¢/
is expected to be approximately real in a CPT-invariant theory [14]. A key prediction of the KM theory is that
¢/
should be a number of order 10-3. Two types of amplitudes contribute to K ® pp decays.
1. Tree amplitudes, involving the quark subprocess s ® u
d, have both DI = 1/2 and DI = 3/2 components and thus contribute to both Ipp = 0 and Ipp = 2 states. In a standard convention [21], tree amplitudes contain no weak phases, since they involve the CKM elements Vud and Vus.2. Penguin amplitudes, involving the quark subprocess s ® d with an intermediate loop consisting of a W boson and the quarks u, c, t, and interacting with the rest of the system through one or more gluons, have only DI = 1/2 and thus can only contribute to the Ipp = 0 state. The top quark in the loop gives rise to a weak phase through the CKM element Vtd.
A relative weak phase of Ipp = 0 and Ipp = 2 states is thus generated in the KM theory, leading to ¢/
¹ 0. Electroweak penguin amplitudes, in which the gluon connecting the s ® d subprocess to the rest of the diagram is replaced by a photon or Z0, can have both DI = 1/2 and DI = 3/2 components and tend to reduce the predicted value of
¢/
. One range of estimates [34] finds a broad and somewhat asymmetric probability distribution extending from slightly below zero to above 2 × 10-3. Others (see articles in [35]) permit slightly higher values.
Recent experiments on Re(¢/
) [36, 37, 38, 39] are summarized in Table II. (The error in the average includes a scale factor [31] of 1.86.) The magnitude of
¢/
is consistent with estimates based on the Kobayashi-Maskawa theory. The qualitative agreement is satisfactory, given that we still cannot account reliably for the large enhancement of DI = 1/2 amplitudes with respect to DI = 3/2 amplitudes in CP-conserving K ® pp decays. More data are expected from the Fermilab and CERN experiments, reducing the eventual statistical error on
¢/
to a part in 104.
V.2 K ® pl+l- information
1. The decay K+® p+n involves loop diagrams involving Vtd and a small charm correction in such a way that the combination |1.4 - r - ih| is constrained, with a predicted branching ratio of order
or for the range permitted in Fig. 6, a branching ratio of about (0.8 ± 0.2) × 10-10 [40]. Additional uncertainties are associated with mc [41] and |Vcb|. A measurement of (K+® p+n
) to 10% will help to constrain (r, h) more tightly than in Fig. 6 or will expose inconsistencies in our present picture of CP violation.
Up to now the Brookhaven E787 Collaboration sees only one K+® p+n event with negligible background [42], corresponding to
More data are expected from the final analysis of this experiment, as well as from a future version with improved sensitivity.
2. The decays KL ® p0l+l- should be dominated by CP-violating contributions, both indirect (~ ) and direct, with a CP-conserving "contaminant" from KL ® p0gg ® p0l+l-. The direct contribution probes the parameter h. Each contribution (including the CP-conserving one) is expected to correspond to a p0e+e- branching ratio of a few parts in 1012. However, KL ® p0e+e- may be limited by backgrounds in the gge+e- final state associated with radiation of a photon in KL ® ge+e- from one of the leptons [43]. Present experimental upper limits (90% c.l.) [44] are
still significantly above most theoretical expectations. (See, however, [45].)
3. The decay KL ® p0n should be due entirely to CP violation, and provides a clean probe of h. Its branching ratio, proportional to A4h2, is expected to be about 3 × 10-11. The best current experimental upper limit (90% c.l.) for this process [46] is
(KL ® p0n
) < 5.9 × 10-7, several orders of magnitude above the expected value.
V.3 Other rare kaon decays
1. The decay KL ® p+p-e+e- involves three independent momenta in the final state and thus offers the opportunity to observe a T-odd observable through a characteristic distribution in the angle f between the p+p- and e+e- planes. A CP- or T-violating angular asymmetry in this process has recently been reported [47, 48].
2. The decay KL ® m+m- g has been studied with sufficiently high statistics to permit a greatly improved measurement of the virtual-photon form factor in KL ® g*g [49]. This measurement is useful in estimating the long-distance contribution to the real part of the amplitude in KL ® g(*)g(*)®m+m-, which in turn allows one to limit the short-distance contribution to KL ® m+m-.
V.4 Is the CKM picture of CP violation correct?
The KM theory is comfortable with the observed range of ¢/
, and its prediction for
(K+® p+n
) is consistent with the one event seen so far. Further anticipated tests are the measurement h through the decay KL ® p0n
(see below), and the search for CP violation in hyperon decays, which is already under way [50, 51]. One also looks forward to a rich set of effects in decays of particles containing b quarks, particularly B mesons. We now describe the experiments and the effects they are expected to see.
VI CP violation in B decays
VI.1 Current and planned experiments
Asymmetric e+e- collisions are being studied at "B factories": the PEP-II machine at SLAC with the BaBar detector, and the KEK-B collider in Japan with the Belle detector. By July 2000, these detectors had accumulated about 14 and 6 fb-1 of data at the energy of the U(4S) resonance, which decays almost exclusively to B [52, 53]. As of September, 2000, PEP-II and KEK-B were providing about 150 and 100 pb-1 per day to their respective detectors.
Further data on e+e- collisions at the (4S) will be provided by the Cornell Electron Storage Ring with the upgraded CLEO-III detector. The HERA-b experiment at DESY in Hamburg hopes to study b quark production via the collisions of 920 GeV protons with a fixed target. The CDF and D0 detectors at Fermilab will devote a significant part of their program at Run II of the Tevatron to B physics. One can expect further results on B physics from the general-purpose LHC detectors ATLAS and CMS, and the dedicated detectors at LHC-b at CERN and BTeV at Fermilab.
VI.2 Types of CP violation
In contrast to neutral kaons, whose mass eigenstates differ in lifetime by nearly a factor of 600, the corresponding B0-0 mass eigenstates are predicted to differ in lifetime by at most 10-20% for strange B's [54, 55], and much less for nonstrange B's. Thus, instead of mass eigenstates like KL, two main types of B decays are of interest: decays to CP eigenstates, and "self-tagging" decays. Both have their advantages and disadvantages.
1. Decays to CP eigenstates f = ±CP(f) utilize interference between direct decays B0® f or 0® f and the corresponding paths involving mixing: B0®
0® f or
0® B0® f. Final states such as f = J/yKS provide examples in which one quark subprocess is dominant. In this case one measures sin2 b with negligible corrections. For f = p+p-, one would measure sin2 a only if the direct decay were dominated by a "tree" amplitude (the quark subprocess b ® u
2. "Self-tagging" decays involve final states f such as K+p- which can be distinguished from their CP-conjugates . A CP-violating rate asymmetry arises when two weak amplitudes ai with weak phases fi and strong phases di (i=1, 2) interfere:
The weak phase changes sign under CP-conjugation, while the strong phase does not. The rate asymmetry is then
The two amplitudes must have different weak and strong phases in order for a rate asymmetry to be observable. The CKM theory predicts the weak phases, but no reliable estimates of strong phases exist. We shall note some ways to avoid this problem.
VI.3 Decays to CP eigenstates
The interference between direct and mixing terms in B decays to CP eigenstates modulates the exponential decay (see, e.g., [57]):
where the upper sign refers to B0 decays and the lower to 0 decays. Dm is the mass splitting, and l0 expresses the interference of decay and mixing amplitudes. For f = J/yKS, l0 = -e-2ib, while for f = p+p-, l0
where x º Dm/G. This expression is maximum for x = 1, and 96% of maximum for the observed value x 0.76.
The CDF Collaboration [58] "tags" neutral B mesons at the time of their production and measures the decay rate asymmetry in B0 (0) ® J/yKS. This asymmetry arises from the phase 2b characterizing the two powers of Vtd in the B0-
0 mixing amplitude. The tagging methods are of two main types. In "opposite-side" methods, since strong interactions produce b and
in pairs, one learns the initial flavor of a decaying B from the "other" b-containing hadron produced in association with it. "Same-side" methods [59] utilize the fact that a B0 tends to be associated more frequently with a p+, and a
0 with a p-, somewhere nearby in phase space.
Electron-positron collisions provide B mesons in pairs at the c.m. energy of the (4S) resonance, just above threshold, in states of negative charge-conjugation eigenvalue. It then becomes necessary to distinguish the vertices of the decaying and tagging B's from one another when studying CP eigenstates. If t and t¢ denote the decay and tagging proper times, the asymmetry for decay to a CP eigenstate will be proportional to sinDm (t-t¢), which vanishes when integrated over all times (see, e.g., [24] or [62]). The BaBar and BELLE results were obtained using asymmetric e+e- collisions, with typical vertex separations of about 250 mm and 200 mm. PEP-II, constructed in the ring of the old PEP machine, collides 9 GeV electrons with 2.7 GeV positrons, while KEK-B, constructed in the TRISTAN tunnel, collides 8.5 GeV electrons with 3.5 GeV positrons. In symmetric collisions the U(4S) is produced at rest and the proper path length of a decaying B is only about 30 mm.
Both BaBar and BELLE used tags based on leptons and kaons from B decays. BaBar also used two neural net methods. The samples reported by the summer of 2000 [52, 53] are shown in Table III.
The CDF result and ones from OPAL [60] and ALEPH [61] utilizing B's produced in the decays of the Z0 are compared with those from BaBar and BELLE in Table IV. The average [24] corresponds to the ±1s rays plotted in Fig. 6. There is no contradiction (yet!) with the allowed region, but we look forward eagerly to reduced errors from BaBar and BELLE. New results are due to be presented in February of 2001.
Samples reported in July 2000 by BaBar and BELLE Collaborations relevant to measurement of sin2 b.
VI.4 "Self-tagging" decays
A typical "self-tagging" mode suitable for the study of "direct" CP violation is B0® K+p-. The tree amplitude [Fig. 7(a)] involves the quark subprocess ®
u
with CKM factor
Vus (weak phase g). The penguin amplitude [Fig. 7(b)]
®
with intermediate u, c, t quarks has CKM factor
Vts or
Vcs (weak phase p or 0), depending on how the unitarity of the CKM matrix is used. The relative weak phase between the tree and penguin amplitudes thus is non-zero, and direct CP violation can arise if the relative strong phase dT- dP also is non-zero. The interpretation of a rate difference G(B0® K+p-) ¹ G(
0® K-p+) requires independent information on dT- dP.
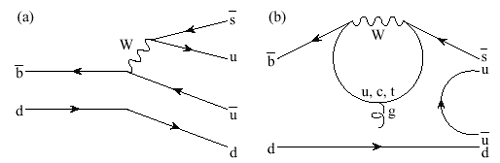


Values of sin 2b implied by recent measurements of the CP-violating asymmetry in B0® J/yKS.
If one measures both a CP-violating asymmetry and a rate ratio such as G(B ® K±p)/G(B±® Kp±) or G(B±® K±p0)/G(B±® Kp±), one can eliminate the strong phase difference and solve for g [63, 64, 65]. One must deal with electroweak penguins (which also affected the interpretation of
¢/
). One proposal (see the first of Refs. [63]) to extract g from the rates for B+® (p0K+, p+K0, p+p0) and the charge-conjugate processes was flawed by the neglect of these contributions, which are important [66]. However, they can be calculated [65], so that measurements of the rates for these processes can yield useful information on g.
A necessary condition for the observability of direct CP asymmetries based on the interference of two amplitudes, one weaker than the other, is that one must be able to detect processes at the level of the absolute square of the weaker amplitude [67]. Let the weak phase difference Df and the strong phase difference Dd both be near ±p/2 (the most favorable case). Then the rate asymmetry in Eq. (31) has magnitude
Define a rate based on the square of each amplitude: Ni = const. |Ai|2. Then ||
2
.
The statistical error in is based on the total number of events. For A2 << A1, one has d
1/
. Then the significance of the asymmetry (in number of standard deviations) is
Thus (aside from the factor of 2) one must be able to see the square of the weaker amplitude at a significant level in order to see a significant asymmetry due to A1-A2 interference.
In searching for direct CP asymmetries one thus considers B decays with at least two amplitudes having an expected weak phase difference, with a large enough rate that the smaller amplitude alone would be detectable, and with a good chance for a strong phase difference.
Many branching ratios for charmless B decays are one to several parts in 105. Rates associated with the subdominant amplitudes are expected to be l2 1/20 of these. Thus when sensitivities to branching ratios of a few parts in 107 are reached, searches for direct CP asymmetries will take on great significance.
Two processes whose rates favor a weak phase g exceeding 90° are B0® p+p- and B0® K*+p- [65, 68, 69], which favor destructive and constructive tree-penguin interference, respectively. A fit to these and other processes in the second of Refs. [69] finds g = (114)°, grazing the allowed region of Fig. 6 but inconsistent with some more restrictive fits [25]. Since the upper bound on g is set by the limit on Bs-
s mixing, Dms > 15 ps-1, such mixing should be visible soon. There is a hint of a signal at ~ 17 ps-1 [29].
The Tevatron and the LHC will produce many neutral B's decaying to p+p-, K±p, and K+K- [70]. Each of these channels has particular advantages.
1. The decays B0® K+K- and Bs ® p+p- should be suppressed unless these final states are "fed" by rescattering from other channels [71].
2. The decays B0® p+p- and Bs ® K+K- can yield g via time-dependence measurements [72].
3. A recent proposal for measuring g [73] utilizes the decays B0® K+p-, B+® K0p+, Bs ® K-p+, and the corresponding charge-conjugate processes. The B0® K+p- and Bs ® K-p+ peaks are well separated from one another and from B0® p+p- and Bs ® K+K- kinematically [70].
The proposal of Ref. [73] is based on the observation that B ® Kp decays involve tree (T) and penguin (P) amplitudes with relative weak phase g and relative strong phase d. The decays B±® Kp± are expected to be dominated by the penguin amplitude (there is no tree contribution except through rescattering from other final states), so this channel is not expected to display any CP-violating asymmetries. The prediction G(B+® K0p+) = G(B-® 0p-) thus will check the assumption that rescattering effects can be neglected. A typical amplitude is given by A(B0 ® K+p-) = - [P + T ei(g+ d)], where the signs are associated with phase conventions for states [74]. Defining
and r º T/P, º Vus/Vud, one finds
The sum of R and Rs allows one to determine r. Using R, r, and A0, one can solve for both d and g. The prediction As = - A0 checks the flavor SU(3) assumption on which these relations are based. An error of 10° on g seems feasible with forthcoming Tevatron data.
Recent upper limits on CP-violating asymmetries in B decays to light-quark systems [75], defined as
are shown in Table V. No significant asymmetries have been seen, but sensitivities adequate to check the maximum predicted values [76] || £ 1/3 are being approached.
VII The role of charm
VII.1 Mixing and CP violation
The dominant decay modes of the neutral charmed mesons D0 and are to states of negative and positive strangeness, respectively, and not to CP eigenstates. Thus D0-
mixing induced by shared final states is expected to be small. Short-distance contributions to mixing also are expected to be small. Thus, in contrast to the case of neutral kaons and B mesons, one expects small mass splittings, Dm/G << 1, and, in contrast to neutral kaons, also small width differences. The degree to which cancellations among contributions of intermediate states such as p+p-, K+K-, and K±p
to mixing suppress such effects further is a matter of debate [77]. If any rate difference is expected, it would be in the direction favoring a slightly greater rate for the CP-even mass eigenstate.
CP violation in the charm sector is expected to be small in the Standard Model. It is also easy to look for, since D mesons are easier to produce than B mesons and the Standard Model background is low.
Recent interesting studies of mixing by the CLEO [78] and FOCUS [79] Collaborations hint at the possibility of non-zero values of Dm, DG, or both, but are not yet statistically compelling. No evidence for mixing is found by the Fermilab E791 Collaboration [80]. It may be necessary to invoke large final-state phase differences in order to reconcile the CLEO and FOCUS results [81]. No CP-violating asymmetries have been seen in charmed meson decays at the level of several percent [80, 82].
VII.2 Spectroscopy
A wide variety of excited cqq and c states are accessible at CLEO and FOCUS. The cqq states are providing unique insights into baryon spectroscopy [83, 84, 85], while the c
states [86, 87], are important sources of information about the corresponding b
states, useful for "same-side" tagging of neutral B mesons.
VIII The future
VIII.1 Envisioned measurements
Future CP studies involve a broad program of experiments with kaons, charmed and B mesons, and neutrinos.
1. Rare kaon decays: Measurement of the branching ratio for KL ® p0n at the required sensitivity (
3 × 10-3) is foreseen at Brookhaven National Laboratory [88] and the Fermilab Main Injector [89]. A Fermilab proposal [90] seeks to acquire enough events of K+® p+n
to measure |Vtd| to a precision of 10%.
2. Charmed mesons: While great strides have been taken in the measurement of mass and lifetime differences for CP eigenstates of the neutral charmed mesons D0, [78, 79], it would be worth while to follow up present hints of nonzero effects. Both electron-positron colliders and hadronic experiments devoted to future B studies may also have more to say about mixing, lifetime differences, and CP violation for charmed mesons.
3. B production in symmetric e+e- collisions: Although asymmetric e+e- colliders are now taking data at an impressive rate, the CLEO Collaboration is continuing with an active program. It will be able to probe charmless B decays down to branching ratios of 10-6. It may be able to detect the elusive B0® p0p0 mode, whose rate will help pin down the penguin amplitude's contribution and permit a determination of the CKM phase a [56]. Other final states of great interest at this level include VP and VV, where P,V denote light pseudoscalar and vector mesons. A useful probe of rescattering effects [71] is the decay B0® K+K-. This decay is expected to have a branching ratio of only a few parts in 108 if rescattering is unimportant, but could be enhanced by more than an order of magnitude in the presence of rescattering from other channels. A challenging but crucial channel is B+® t+
t, whose rate will provide information on the combination fB|Vcb|. Rare decays such as B ® X

4. B production in asymmetric e+e- collisions: The BaBar and Belle detectors have made a start at the measurement of sin2 b in B0® J/yKS. The moving center-of-mass facilitates both flavor tagging and improvement of signal with respect to background. These machines will make possible a host of time-dependent studies in such decays as B ®pp, B ® Kp, etc., and their impressive luminosities will eventually add significantly to the world's tally of detected B's.
5. Hadronic B production: The strange B's cannot be produced at the (4S) which will dominate the attention of e+e- colliders for some years to come. Hadronic reactions at high energies will produce copious b's incorporated into nonstrange, strange, and charmed mesons, and baryons. A measurement of the strange-B mixing parameter Dmsis likely to be made soon. Bs decays provide valuable information on CKM phases and CP violation, as in Bs ® K+K- [72]. The width difference expected between the CP-even and CP-odd eigenstates of the Bs system [54, 55] should be visible in the next round of experiments.
6. Neutrino studies: The magnitudes and phases in the CKM matrix are connected with the quark masses themselves, whose pattern we will not understand until we have mapped out a similar pattern for the leptons. We will learn much about neutrino masses and mixings from forthcoming experiments at the Sudbury Neutrino Observatory [91], Borexino [92], K2K [93], and Fermilab (BooNE and MINOS) [94].
VIII.2 A likely parameter space
Our knowledge of the Cabibbo-Kobayashi-Maskawa is likely to improve over the next few years [95, 96]. With sin(2 b) measured in B0® J/yKS decays to an accuracy of ±0.06 (the BaBar goal with 30 fb-1 [62]), errors on |Vub/Vcb| reduced to 10%, strange-B mixing bounded by xs = Dms/Gs > 20 (the present bound is already better than this!), and (B+® t+nt) measured to ±20% (giving fB|Vub|, or |Vub/Vtd| when combined with B0-
0 mixing), one finds the result shown in Fig. 8.
Plot in (r, h) of anticipated constraints on CKM parameters in the year 2003. Solid curves: |Vub/Vcb|; dashed lines: constraint on |Vub/Vtd| by combining measurement of
The narrow range of (r, h) increases the chance that any non-standard physics will show up as a contradiction among various measurements, most likely by providing additional contributions to B0-0 mixing [97] but possibly directly affecting decays [98].
VIII.3 Baryon number of the Universe
The number of baryons in the Universe is much larger than the corresponding number of antibaryons. Sakharov proposed [99] three requirements for this preponderance of matter over antimatter: (1) an epoch in which the Universe was not in thermal equilibrium, (2) an interaction violating baryon number, and (3) CP (and C) violation. The observed baryon asymmetry is not explained directly by the CP violation in the CKM matrix; the effects are too small, requiring some new physics. Two examples are the following:
1. Supersymmetry, in which each particle of spin J has a "superpartner" of spin J ± 1/2, affords many opportunities for introducing new CP-violating phases and interactions which could affect particle-antiparticle mixing [100].
2. Neutrino masses at the sub-eV level can signal large right-handed neutrino Majorana masses, exceeding 1011 GeV [101]. Lepton number (L), violated by such masses, can be reprocessed into baryon number (B) by B-L conserving interactions at the electroweak scale [102]. New CP-violating interactions must exist at the high mass scale if lepton number is to be generated there. These interactions could be related to CKM phases [103]. If this alternative is correct, it will be important to understand the leptonic analogue of the CKM matrix!
VIII.4 Surprises ahead?
The CKM theory of CP violation in neutral kaon decays has passed a crucial test. The parameter ¢/
is nonzero, and has the expected order of magnitude. Tests using B mesons, including the observation of a difference in rates between B0® J/yKS and
0 ® J/yKS, are just around the corner. Progress in "tagging" neutral B's and rich information from measurements of many B decay rates will round out the picture.
If B decays do not provide a consistent set of CKM phases in the next few years, we will re-examine other proposed sources of CP violation. Most of these, in contrast to the CKM theory, predict neutron and electron dipole moments very close to their present experimental upper limits. If, however, the CKM picture remains self-consistent, we should ask about the origin of the CKM phases and the associated quark and lepton masses. It is probably time to start anticipating this possibility, given the resilience of the CKM picture since it was first proposed nearly 30 years ago.
I am looking forward to a surprise such as one encountered many years ago when exploring a small cave in Pennsylvania. We had entered it in the afternoon and thought we had seen all its rooms, when I came upon another chamber with ghostly stalactites silhouetted against the darkness behind them. A breeze of warm air signaled that I was actually looking outside, with the "stalactites" the faintly glowing night sky, and the dark spaces the shadows of pine trees. Such a "perception shift" does not come often, but is a welcome source of wonder.
Acknowledgments
It is a pleasure to thank Adriano Natale and Rogerio Rosenfeld for the invitation to attend the Brazilian Meeting of Particles and Fields and for their wonderful hospitality in Sao Paulo and Sao Lourenço, and Patrick Roudeau for constructive correspondence. This work was supported in part by the United States Department of Energy under Grant No. DE FG02 90ER40560.
References
[1] J. L. Rosner, in Particle Physics and Cosmology: 2nd Tropical Workshop, edited by Jose F. Nieves (New York, American Institute of Physics, 2000), pp. 283-304.
[2] J. H. Christenson, J. W. Cronin, V. L. Fitch, and R. Turlay, Phys. Rev. Lett. 13, 138-140 (1964).
[3] J. Schwinger, Phys. Rev. 91, 713-728 (1953); Phys. Rev. 94, 1362-1384 (1954); G. Lüders, Kong. Danske Vid. Selsk., Matt-fys. Medd. 28, No. 5, 1-17 (1954); Ann. Phys. (N.Y.) 2, 1-15 (1957); W. Pauli, in Niels Bohr and the Development of Physics, edited by W. Pauli (Pergamon, New York, 1955), pp. 30-51.
[4] M. Kobayashi and T. Maskawa, Prog. Theor. Phys. 49, 652-657 (1973).
[5] N. Cabibbo, Phys. Rev. Lett. 10, 531-532 (1963).
[6] S. M. Barr, in TASI 94: CP Violation and the Limits of the Standard Model, Boulder, CO, 29 May - 24 June 1994, edited by J. F. Donoghue (World Scientific, River Edge, NJ, 1995), pp. 87-111.
[7] G. D. Rochester and C. C. Butler, Nature 160, 855-857 (1947).
[8] M. Gell-Mann, Phys. Rev. 92, 833-834 (1953); " On the Classification of Particles," 1953 (unpublished); M. Gell-Mann and A. Pais, in Proceedings of the 1954 Glasgow Conference on Nuclear and Meson Physics, edited by E. H. Bellamy and R. G. Moorhouse (Pergamon, London and New York, 1955); M. Gell-Mann, Nuovo Cim. 4, Suppl. 848-866 (1956); T. Nakano and K. Nishijima, Prog. Theor. Phys. 10, 581-582 (1953); K. Nishijima, Prog. Theor. Phys. 12, 107-108 (1954); Prog. Theor. Phys. 13, 285-304 (1955).
[9] M. Gell-Mann and A. Pais, Phys. Rev. 97, 1387-1389 (1955).
[10] K. Lande, E. T. Booth, J. Impeduglia, and L. M. Lederman, Phys. Rev. 103, 1901-1904 (1956).
[11] T. D. Lee, R. Oehme, and C. N. Yang, Phys. Rev. 106, 340-345 (1957); L. D. Landau, Nucl. Phys. 3, 127-131 (1957).
[12] B. Winstein, in Festi-Val - Festschrift for Val Telegdi, ed. by K. Winter (Elsevier, Amsterdam, 1988), pp. 245-265.
[13] L. Wolfenstein, Phys. Rev. Lett. 13, 562-564 (1964).
[14] For reviews, see, e.g., R. G. Sachs, The Physics of Time Reversal Invariance (University of Chicago Press, Chicago, 1988); K. Kleinknecht, in CP Violation, edited by C. Jarlskog (World Scientific, Singapore, 1989)89, pp. 41-104; J. L. Rosner, in Testing the Standard Model (Proceedings of the 1990 Theoretical Advanced Study Institute in Elementary Particle Physics, Boulder, Colorado, 3-27 June, 1990), edited by M. Cvetic and P. Langacker (World Scientific, Singapore, 1991), pp. 91-224; B. Winstein and L. Wolfenstein, Rev. Mod. Phys. 63, 1113-1148 (1992); G. C. Branco, L. Lavoura, and J. P. Silva, CP Violation (Oxford, 1999); I. I. Bigi and A. I. Sanda, CP Violation (Cambridge, 2000).
[15] J. L. Rosner, Am. J. Phys. 64, 982-985 (1996).
[16] V. A. Kostelecký and Á. Roberts, Phys. Rev. D 63, 096002 (2001).
[17] J. L. Rosner and S. A. Slezak, Am. J. Phys. 69, 44-49 (2001).
[18] J. D. Bjorken and S. L. Glashow, Phys. Lett. 11, 255-257 (1964); Y. Hara, Phys. Rev. 134, B701-B704 (1964); Z. Maki and Y. Ohnuki, Prog. Theor. Phys. 32, 144-158 (1964); S. L. Glashow, J. Iliopoulos, and L. Maiani, Phys. Rev. D 2, 1285-1292 (1970).
[19] Fermilab E288 Collaboration, S. W. Herb et al., Phys. Rev. Lett. 39, 252-255 (1977); W. R. Innes et al., Phys. Rev. Lett. 39, 1240-1242,1640(E) (1977).
[20] CDF Collaboration, F. Abe et al., Phys. Rev. D 50, 2966-3026 (1944); 51, 4623-4637 (1994); 52, 2605-2609 (1995); Phys. Rev. Lett. 73, 225-231 (1994); 74, 2626-2631 (1995); D0 Collaboration, S. Abachi et al., Phys. Rev. Lett. 72, 2138-2142 (1994); 74, 2422-2426 (1995); 74, 2632-2637 (1995); Phys. Rev. D 52, 4877-4919 (1995).
[21] L. Wolfenstein, Phys. Rev. Lett. 51, 1945-1947 (1983).
[22] M. Gell-Mann and M. Lévy, Nuovo Cim. 16, 705-725 (1960).
[23] J. L. Rosner, Enrico Fermi Institute Report No. EFI 2000-42, hep-ph/0011184. To be published in Proceedings of Beauty 2000, Kibbutz Maagan, Israel, September 13-18, 2000, edited by S. Erhan, Y. Rozen, and P. E. Schlein, Nucl. Inst. Meth. A, 2001.
[24] J. L. Rosner, Enrico Fermi Institute Report No. EFI 2000-47, hep-ph/0011355. To be published in Flavor Physics for the Millennium (Proceedings of the TASI-2000 Summer School, Boulder, CO, June 5-30, 2000), edited by J. L. Rosner (World Scientific, Singapore, 2001).
[25] F. Gilman, K. Kleinknecht, and Z. Renk, mini-review on pp. 110-114 of Particle Data Group, C. Caso et al., Eur. Phys. J. C 15, 1-878 (2000); A. Ali and D. London, DESY report DESY-00-026, hep-ph/0002167, in Proceedings of the 3rd Workshop on Physics and Detectors for DAPHNE, Frascati, Italy, Nov. 16-19, 1999, edited by S. Bianco et al. (INFN, 1999), pp. 3-23; S. Stone, Conference Summary, Beauty 2000, hep-ex/0012162, to be published in Proceedings of Beauty 2000 [23]; M. Ciuchini et al., Orsay preprint LAL 00-77, hep-ex/0012308, to be submitted to JHEP.
[26] CLEO Collaboration, J. P. Alexander et al., CLEO-CONF 00-3, presented at Osaka Conference XXX International Conference on High Energy Physics, Osaka, Japan, July 27 - August 2, 2000.
[27] A. F. Falk, inProceedings of the XIXth International Symposium on Lepton and Photon Interactions, Stanford, California, August 9-14 1999, edited by J. Jaros and M. Peskin (World Scientific, Singapore, 2000), Electronic Conference Proceedings C990809, 174 (2000).
[28] C. Sachrajda, to be published in Proceedings of Beauty 2000 [23].
[29] D. Abbaneo, presented at Conference on Heavy Quarks at Fixed Target, Rio de Janeiro, Oct. 9-19, 2000, hep-ex/0012010. Current world averages for nonstrange and strange neutral B mixing amplitudes may be found in http://www.cern.ch/LEPBOSC/.
[30] J. L. Rosner, Phys. Rev. D 42, 3732-3740 (1990).
[31] Particle Data Group, C. Caso et al., Eur. Phys. J. C 15, 1-878 (2000).
[32] V. Lubicz, Invited Talk at the XX Physics in Collision Conference, June 29 - July 1, 2000, Lisbon, Portugal, Univ. of Rome III report RM3-TH/00-15, hep-ph/0010171.
[33] Bose statistics forbid a spinless pp state with I=1.
[34] A. J. Buras, M. Jamin, and M. E. Lautenbacher, Phys. Lett. B 389, 749-756 (1996).
[35] See the articles on ¢/
by A. J. Buras, S. Bertolini, R. Gupta, G. Martinelli, and W. A. Bardeen in Kaon Physics, edited by J. L. Rosner and B. Winstein (University of Chicago Press, 2001).
[36] Fermilab E731 Collaboration, L. K. Gibbons et al., Phys. Rev. Lett. 70, 1203-1206 (1993); Phys. Rev. D 55, 6625-6715 (1997).
[37] CERN NA31 Collaboration, G. D. Barr et al., Phys. Lett. B 317, 233-242 (1993).
[38] Fermilab E832/KTeV Collaboration, A. Alavi-Harati et al., Phys. Rev. Lett. 83, 22-27 (1999).
[39] NA48 Collaboration, G. D. Barr et al., presented at CERN seminar by A. Ceccucci, Feb. 29, 2000 (unpublished). Follow the links on http://www/cern.ch/NA48/ for a copy of the transparencies.
[40] G. Buchalla and A. J. Buras, Nucl. Phys. B548, 309-327 (1999); G. Buchalla, in Kaon Physics [35].
[41] A. F. Falk, A. Lewandowski, and A. A. Petrov, Cornell Univ. report CLNS-00-1707, hep-ph/0012099.
[42] Brookhaven E787 Collaboration, S. Adler et al., Phys. Rev. Lett. 84, 3768-3770 (2000).
[43] H. B. Greenlee, Phys. Rev. D 42, 3724-3731 (1990).
[44] Fermilab E-799-II/KTeV Collaboration, A. Alavi-Harati et al., preprint hep-ex/0011093 (p0e+e-), Phys. Rev. Lett. 84, 5279-8282 (2000) (p0m+m-).
[45] P. Ko, Phys. Rev. D 44, 139-165 (1991).
[46] Fermilab E-799-II/KTeV Collaboration, A. Alavi-Harati et al., Phys. Rev. D 61, 072006 (2000).
[47] Fermilab E-799-II/KTeV Collaboration, A. Alavi-Harati et al., Phys. Rev. Lett. 84, 408-411 (2000).
[48] NA48 Collaboration, A. Lai et al., Phys. Lett. B 496, 137-144 (2000).
[49] KTeV Collaboration, G. Breese Quinn, Ph. D. Thesis, University of Chicago, May, 2000 (unpublished).
[50] CLEO Collaboration, D. E. Jaffe et al., Cornell University report CLNS-98-1587, hep-ex/0009037 (unpublished).
[51] Fermilab E756 Collaboration, K. B. Luk et al., Phys. Rev. Lett. 85, 4860 (2000).
[52] BaBar Collaboration, reported by D. Hitlin at Osaka Conf. [26], hep-ex/0011024.
[53] BELLE Collaboration, reported by H. Aihara at Osaka Conf. [26], hep-ex/0010008.
[54] M. Beneke, G. Buchalla, and I. Dunietz, Phys. Rev. D 54, 4419-4431 (1996).
[55] D. Becirevic et al., Eur. Phys. J. C 18, 157-166 (2000).
[56] M. Gronau and D. London, Phys. Rev. Lett. 65, 3381-3384 (1990).
[57] I. Dunietz and J. L. Rosner, Phys. Rev. D 34, 1404-1417 (1986).
[58] CDF Collaboration, T. Affolder et al., Phys. Rev. D 61, 072005 (2000).
[59] M. Gronau, A. Nippe, and J. L. Rosner, Phys. Rev. D 47, 1988-1993 (1993); M. Gronau and J. L. Rosner, Phys. Rev. Lett. 72, 195-198 (1994); Phys. Rev. D 49, 254-264 (1994); Phys. Rev. D 63, 054006 (2001).
[60] OPAL Collaboration, K. Ackerstaff et al., Eur. Phys. J. C 5, 379-388 (()1998).
[61] ALEPH Collaboration, R. Barate et al., Phys. Lett. B 492, 259-274 (2000).
[62] The BaBar Physics Book: Physics at an Asymmetric B Factory, edited by P. F. Harrison and H. R. Quinn, SLAC Report SLAC-504, 1998.
[63] M. Gronau, J. Rosner and D. London, Phys. Rev. Lett. 73, 21-24 (1994); M. Gronau and J. L. Rosner, Phys. Rev. Lett. 76, 1200-1203 (1996); Phys. Rev. D 57, 6843-6850 (1998); A. S. Dighe, M. Gronau, and J. L. Rosner, Phys. Rev. D 54, 3309-3320 (1996); A. S. Dighe and J. L. Rosner, 54, 4677-4679 (1996); M. Gronau and D. Pirjol, Phys. Lett. B 449, 321-327 (1999); Phys. Rev. D 61, 013005 (2000).
[64] R. Fleischer, Phys. Lett. B 365, 399-406 (1996); Phys. Rev. D 58, 093001 (1998); R. Fleischer and T. Mannel, Phys. Rev. D 57, 2752-2759 (1998); A. J. Buras, R. Fleischer, and T. Mannel, Nucl. Phys. B533, 3-24 (1998); R. Fleischer and A. J. Buras, Eur. Phys. J. C 11, 93-109 (1999); A. J. Buras and R. Fleischer, Eur. Phys. J. C 16, 97-104 (2000).
[65] M. Neubert and J. L. Rosner, Phys. Lett. B 441, 403-409 (1998); Phys. Rev. Lett. 81, 5076-5079 (1998); M. Neubert, JHEP 9902, 014 (1999).
[66] N. G. Deshpande and X.-G. He, Phys. Rev. Lett. 74, 26-29 (1995); 74, 4099(E) (1995); O. F. Hernández, D. London, M. Gronau, and J. L. Rosner, Phys. Rev. D 52, 6374-6382 (1995).
[67] G. Eilam, M. Gronau, and J. L. Rosner, Phys. Rev. D 39, 819-824 (1989).
[68] M. Gronau and J. L. Rosner, Phys. Rev. D 61, 073008 (2000).
[69] X.-G. He, W.-S. Hou, and K.-C. Yang, Phys. Rev. Lett. 83, 1100-1103 (1999); W.-S. Hou, J. G. Smith, and F. Würthwein, preprint hep-ex/9910014 (unpublished).
[70] F. Würthwein and R. Jesik, talks for Working Group 1 presented at Workshop on B Physics at the Tevatron - Run II and Beyond, Fermilab, February 2000 (unpublished).
[71] B. Blok, M. Gronau, and J. L. Rosner, Phys. Rev. Lett. 78, 3999-4002 (1997); 79, 1167 (1997); A. Falk, A. L. Kagan, Y. Nir, and A. A. Petrov, Phys. Rev. D 57, 4290-4300 (1998); M. Gronau and J. L. Rosner, Phys. Rev. D 57, 6843-6350 (1998); 58, 113005 (1998); R. Fleischer, Phys. Lett. B 435, 221-232 (1998); Eur. Phys. J. C 6, 451-470 (1999).
[72] R. Fleischer, Phys. Lett. B 459, 306-320 (1999); Eur. Phys. J. C 16, 87-95 (2000). See also I. Dunietz, Proceedings of the Workshop on B Physics at Hadron Accelerators, Snowmass, CO, 1993, pp. 83-96; D. Pirjol, Phys. Rev. D 60, 054020 (1999).
[73] M. Gronau and J. L. Rosner, Phys. Lett. B 482, 71-76 (2000).
[74] M. Gronau, O. F. Hernández, D. London and J. L. Rosner, Phys. Rev. D 50, 4529-4543 (1994).
[75] CLEO Collaboration, S. Chen et al., Phys. Rev. Lett. 85, 525-529 (2000).
[76] M. Gronau and J. L. Rosner, Phys. Rev. D 59, 113002 (1999).
[77] H. Georgi, Phys. Lett. B 297, 353-357 (1992); L. Wolfenstein, Phys. Lett. B 164, 170-172 (1985); Phys. Rev. Lett. 75, 2460-2461 (1995); J. Donoghue, E. Golowich, B. R. Holstein, and J. Trampeti\'c, Phys. Rev. D 33, 179-183 (1986).
[78] CLEO Collaboration, R. Godang et al., Phys. Rev. Lett. 84, 5038-5042 (2000).
[79] FOCUS Collaboration, J. M. Link, et al., Phys. Rev. B 485, 62-70 (2000); Phys. Rev. Lett. 86, 2955-2958 (2001).
[80] Fermilab E791 Collaboration, presented by A. J. Schwartz, Univ. of Cincinnati report UCTP-112-00, hep-ex/0012006 (unpublished); J. A. Appel, hep-ex/0002022, in Proceedings of the 3rd Workshop on Physics and Detectors for DAPHNE [25], pp. 231-247.
[81] A. F. Falk, Y. Nir, and A. A. Petrov, JHEP 12, 019 (1999); S. Bergmann, Y. Grossman, Z. Ligeti, Y. Nir and A. A. Petrov, Phys. Lett. B 486, 418-425 (2000); I. I. Bigi and N. G. Uraltsev, Nucl. Phys. B592, 92-106 (2000).
[82] See, e.g., FOCUS Collaboration, J. M. Link et al., Phys. Lett. B 491, 232-239 (2000); 495, 443(E) (2000).
[83] CLEO Collaboration, D. Cronin-Hennessy et al., Phys. Rev. D 86, 3730-3734 (2001).
[84] CLEO Collaboration, M. Artuso et al., Cornell University report CLNS 00-1698, hep-ex/0010080.
[85] CLEO Collaboration, S. E. Csorna et al., Phys. Rev. 86, 4243-4246 (2001).
[86] CLEO Collaboration, S. Anderson et al., Nucl. Phys. A663, 647-650 (2000).
[87] FOCUS Collaboration, presented by F. L. Fabbri at Osaka Conf. [26], hep-ex/0011044; presented by C. Ricciardi, Nucl. Phys. A663, 651-654 (2000).
[88] KOPIO Collaboration, in Rare Symmetry Violating Processes, proposal to the National Science Foundation, October 1999, and Brookhaven National Laboratory Proposal P926 (unpublished).
[89] KAMI Collaboration, Fermilab Proposal P804 (unpublished).
[90] CKM Collaboration, Fermilab Proposal P905.
[91] SNO Collaboration, presented by R. van de Water in Particle Physics and Cosmology: 2nd Tropical Workshop [1], pp. 193-200.
[92] See the description of this experiment at http://almime.mi.infn.it/
[93] Super-Kamiokande Collaboration, presented by M. Vagins in Particle Physics and Cosmology: 2nd Tropical Workshop [1], pp. 122-192.
[94] NuTeV Collaboration, presented by J. Conrad in Particle Physics and Cosmology: 2nd Tropical Workshop [1], pp. 331-340; M. Shaevitz, ibid., pp. 105-121.
[95] J. L. Rosner, Nucl. Phys. B Proc. Suppl. 73, 29-42 (1999).
[96] P. Burchat et al., Report of the NSF Elementary Particle Physics Special Emphasis Panel on B Physics, July, 1998 (unpublished).
[97] See, e.g., C. O. Dib, D. London, and Y. Nir, Int. J. Mod. Phys. A 6, 1253-1266 (1991); M. Gronau and D. London, Phys. Rev. D 55, 2845-2861 (1997).
[98] Y. Grossman and M. P. Worah, Phys. Lett. B 395, 241-249 (1997); Y. Grossman, G. Isidori, and M. P. Worah, Phys. Rev. D 58, 057504 (1998).
[99] A. D. Sakharov, Pis'ma Zh. Eksp. Teor. Fiz. 5, 32-35 (1967) [JETP Lett. 5, 24-27 (1967)].
[100] M. P. Worah, Phys. Rev. D 56, 2010-2018 (1997); Phys. Rev. Lett. 79, 3810-3813 (1997); M. Carena, M. Quiros, A. Riotto, I. Vilja, and C. E. M. Wagner, Nucl. Phys. B503, 387-404 (1997); M. P. Worah, in Kaon Physics [35].
[101] P. Ramond, in Particle Physics and Cosmology: 2nd Tropical Workshop [1], pp. 75-90.
[102] G. 't Hooft, Phys. Rev. Lett. 37, 8-11 (1976); M. Fukugita and T. Yanagida, Phys. Lett. B 174, 45-47 (1986); M. A. Luty, Phys. Rev. D 45, 455-465 (1992); M. Plümacher, Zeit. Phys. C 74, 549-559 (1997); W. Buchmüller and M. Plumacher, Phys. Lett. B 389, 73-77 (1996); 431, 354-362 (1998); Phys. Rep. 320, 329-339 (1999).
[103] J. L. Rosner, in The Albuquerque Meeting (Proceedings of the 8th Meeting, Division of Particles and Fields of the American Physical Society, Aug. 2-6, 1994, The University of New Mexico), edited by S. Seidel (World Scientific, Singapore, 1995), pp. 321-350; M. P. Worah, Phys. Rev. D 53, 3902-3912 (1996).
- [1] J. L. Rosner, in Particle Physics and Cosmology: 2nd Tropical Workshop, edited by Jose F. Nieves (New York, American Institute of Physics, 2000), pp. 283-304.
- [2] J. H. Christenson, J. W. Cronin, V. L. Fitch, and R. Turlay, Phys. Rev. Lett. 13, 138-140 (1964).
- [3] J. Schwinger, Phys. Rev. 91, 713-728 (1953);
- Phys. Rev. 94, 1362-1384 (1954);
- G. Lüders, Kong. Danske Vid. Selsk., Matt-fys. Medd. 28, No. 5, 1-17 (1954);
- Ann. Phys. (N.Y.) 2, 1-15 (1957);
- W. Pauli, in Niels Bohr and the Development of Physics, edited by W. Pauli (Pergamon, New York, 1955), pp. 30-51.
- [4] M. Kobayashi and T. Maskawa, Prog. Theor. Phys. 49, 652-657 (1973).
- [5] N. Cabibbo, Phys. Rev. Lett. 10, 531-532 (1963).
- [6] S. M. Barr, in TASI 94: CP Violation and the Limits of the Standard Model, Boulder, CO, 29 May - 24 June 1994, edited by J. F. Donoghue (World Scientific, River Edge, NJ, 1995), pp. 87-111.
- [7] G. D. Rochester and C. C. Butler, Nature 160, 855-857 (1947).
- [8] M. Gell-Mann, Phys. Rev. 92, 833-834 (1953); "
- On the Classification of Particles," 1953 (unpublished);
- M. Gell-Mann and A. Pais, in Proceedings of the 1954 Glasgow Conference on Nuclear and Meson Physics, edited by E. H. Bellamy and R. G. Moorhouse (Pergamon, London and New York, 1955);
- M. Gell-Mann, Nuovo Cim. 4, Suppl. 848-866 (1956);
- T. Nakano and K. Nishijima, Prog. Theor. Phys. 10, 581-582 (1953);
- K. Nishijima, Prog. Theor. Phys. 12, 107-108 (1954);
- Prog. Theor. Phys. 13, 285-304 (1955).
- [9] M. Gell-Mann and A. Pais, Phys. Rev. 97, 1387-1389 (1955).
- [10] K. Lande, E. T. Booth, J. Impeduglia, and L. M. Lederman, Phys. Rev. 103, 1901-1904 (1956).
- [11] T. D. Lee, R. Oehme, and C. N. Yang, Phys. Rev. 106, 340-345 (1957);
- L. D. Landau, Nucl. Phys. 3, 127-131 (1957).
- [12] B. Winstein, in Festi-Val - Festschrift for Val Telegdi, ed. by K. Winter (Elsevier, Amsterdam, 1988), pp. 245-265.
- [13] L. Wolfenstein, Phys. Rev. Lett. 13, 562-564 (1964).
- [14] For reviews, see, e.g., R. G. Sachs, The Physics of Time Reversal Invariance (University of Chicago Press, Chicago, 1988);
- K. Kleinknecht, in CP Violation, edited by C. Jarlskog (World Scientific, Singapore, 1989)89, pp. 41-104;
- J. L. Rosner, in Testing the Standard Model (Proceedings of the 1990 Theoretical Advanced Study Institute in Elementary Particle Physics, Boulder, Colorado, 3-27 June, 1990), edited by M. Cvetic and P. Langacker (World Scientific, Singapore, 1991), pp. 91-224;
- B. Winstein and L. Wolfenstein, Rev. Mod. Phys. 63, 1113-1148 (1992);
- G. C. Branco, L. Lavoura, and J. P. Silva, CP Violation (Oxford, 1999);
- I. I. Bigi and A. I. Sanda, CP Violation (Cambridge, 2000).
- [15] J. L. Rosner, Am. J. Phys. 64, 982-985 (1996).
- [16] V. A. Kostelecký and Á. Roberts, Phys. Rev. D 63, 096002 (2001).
- [17] J. L. Rosner and S. A. Slezak, Am. J. Phys. 69, 44-49 (2001).
- [18] J. D. Bjorken and S. L. Glashow, Phys. Lett. 11, 255-257 (1964);
- Y. Hara, Phys. Rev. 134, B701-B704 (1964);
- Z. Maki and Y. Ohnuki, Prog. Theor. Phys. 32, 144-158 (1964);
- S. L. Glashow, J. Iliopoulos, and L. Maiani, Phys. Rev. D 2, 1285-1292 (1970).
- [19] Fermilab E288 Collaboration, S. W. Herb et al., Phys. Rev. Lett. 39, 252-255 (1977);
- W. R. Innes et al., Phys. Rev. Lett. 39, 1240-1242,1640(E) (1977).
- [20] CDF Collaboration, F. Abe et al., Phys. Rev. D 50, 2966-3026 (1944); 51, 4623-4637 (1994); 52, 2605-2609 (1995);
- Phys. Rev. Lett. 73, 225-231 (1994); 74, 2626-2631 (1995);
- D0 Collaboration, S. Abachi et al., Phys. Rev. Lett. 72, 2138-2142 (1994); 74, 2422-2426 (1995); 74, 2632-2637 (1995);
- Phys. Rev. D 52, 4877-4919 (1995).
- [21] L. Wolfenstein, Phys. Rev. Lett. 51, 1945-1947 (1983).
- [22] M. Gell-Mann and M. Lévy, Nuovo Cim. 16, 705-725 (1960).
- [23] J. L. Rosner, Enrico Fermi Institute Report No. EFI 2000-42, hep-ph/0011184. To be published in Proceedings of Beauty 2000, Kibbutz Maagan, Israel, September 13-18, 2000, edited by S. Erhan, Y. Rozen, and P. E. Schlein, Nucl. Inst. Meth. A, 2001.
- [24] J. L. Rosner, Enrico Fermi Institute Report No. EFI 2000-47, hep-ph/0011355. To be published in Flavor Physics for the Millennium (Proceedings of the TASI-2000 Summer School, Boulder, CO, June 5-30, 2000), edited by J. L. Rosner (World Scientific, Singapore, 2001).
- [25] F. Gilman, K. Kleinknecht, and Z. Renk, mini-review on pp. 110-114 of Particle Data Group, C. Caso et al., Eur. Phys. J. C 15, 1-878 (2000);
- A. Ali and D. London, DESY report DESY-00-026, hep-ph/0002167, in Proceedings of the 3rd Workshop on Physics and Detectors for DAPHNE, Frascati, Italy, Nov. 16-19, 1999, edited by S. Bianco et al. (INFN, 1999), pp. 3-23;
- S. Stone, Conference Summary, Beauty 2000, hep-ex/0012162, to be published in Proceedings of Beauty 2000 [23];
- M. Ciuchini et al., Orsay preprint LAL 00-77, hep-ex/0012308, to be submitted to JHEP.
- [26] CLEO Collaboration, J. P. Alexander et al., CLEO-CONF 00-3, presented at Osaka Conference XXX International Conference on High Energy Physics, Osaka, Japan, July 27 - August 2, 2000.
- [27] A. F. Falk, inProceedings of the XIXth International Symposium on Lepton and Photon Interactions, Stanford, California, August 9-14 1999, edited by J. Jaros and M. Peskin (World Scientific, Singapore, 2000), Electronic Conference Proceedings C990809, 174 (2000).
- [28] C. Sachrajda, to be published in Proceedings of Beauty 2000 [23].
- [29] D. Abbaneo, presented at Conference on Heavy Quarks at Fixed Target, Rio de Janeiro, Oct. 9-19, 2000, hep-ex/0012010. Current world averages for nonstrange and strange neutral B mixing amplitudes may be found in http://www.cern.ch/LEPBOSC/
- [30] J. L. Rosner, Phys. Rev. D 42, 3732-3740 (1990).
- [31] Particle Data Group, C. Caso et al., Eur. Phys. J. C 15, 1-878 (2000).
- [32] V. Lubicz, Invited Talk at the XX Physics in Collision Conference, June 29 - July 1, 2000, Lisbon, Portugal, Univ. of Rome III report RM3-TH/00-15, hep-ph/0010171.
- [34] A. J. Buras, M. Jamin, and M. E. Lautenbacher, Phys. Lett. B 389, 749-756 (1996).
- [35] See the articles on ˘/ by A. J. Buras, S. Bertolini, R. Gupta, G. Martinelli, and W. A. Bardeen in Kaon Physics, edited by J. L. Rosner and B. Winstein (University of Chicago Press, 2001).
- [36] Fermilab E731 Collaboration, L. K. Gibbons et al., Phys. Rev. Lett. 70, 1203-1206 (1993);
- Phys. Rev. D 55, 6625-6715 (1997).
- [37] CERN NA31 Collaboration, G. D. Barr et al., Phys. Lett. B 317, 233-242 (1993).
- [38] Fermilab E832/KTeV Collaboration, A. Alavi-Harati et al., Phys. Rev. Lett. 83, 22-27 (1999).
- [39] NA48 Collaboration, G. D. Barr et al., presented at CERN seminar by A. Ceccucci, Feb. 29, 2000 (unpublished).
- [40] G. Buchalla and A. J. Buras, Nucl. Phys. B548, 309-327 (1999);
- [41] A. F. Falk, A. Lewandowski, and A. A. Petrov, Cornell Univ. report CLNS-00-1707, hep-ph/0012099.
- [42] Brookhaven E787 Collaboration, S. Adler et al., Phys. Rev. Lett. 84, 3768-3770 (2000).
- [43] H. B. Greenlee, Phys. Rev. D 42, 3724-3731 (1990).
- [44] Fermilab E-799-II/KTeV Collaboration, A. Alavi-Harati et al., preprint hep-ex/0011093 (p0 e+ e-), Phys. Rev. Lett. 84, 5279-8282 (2000) (p0m+ m-).
- [45] P. Ko, Phys. Rev. D 44, 139-165 (1991).
- [46] Fermilab E-799-II/KTeV Collaboration, A. Alavi-Harati et al., Phys. Rev. D 61, 072006 (2000).
- [47] Fermilab E-799-II/KTeV Collaboration, A. Alavi-Harati et al., Phys. Rev. Lett. 84, 408-411 (2000).
- [48] NA48 Collaboration, A. Lai et al., Phys. Lett. B 496, 137-144 (2000).
- [49] KTeV Collaboration, G. Breese Quinn, Ph. D. Thesis, University of Chicago, May, 2000 (unpublished).
- [50] CLEO Collaboration, D. E. Jaffe et al., Cornell University report CLNS-98-1587, hep-ex/0009037 (unpublished).
- [51] Fermilab E756 Collaboration, K. B. Luk et al., Phys. Rev. Lett. 85, 4860 (2000).
- [54] M. Beneke, G. Buchalla, and I. Dunietz, Phys. Rev. D 54, 4419-4431 (1996).
- [55] D. Becirevic et al., Eur. Phys. J. C 18, 157-166 (2000).
- [56] M. Gronau and D. London, Phys. Rev. Lett. 65, 3381-3384 (1990).
- [57] I. Dunietz and J. L. Rosner, Phys. Rev. D 34, 1404-1417 (1986).
- [58] CDF Collaboration, T. Affolder et al., Phys. Rev. D 61, 072005 (2000).
- [59] M. Gronau, A. Nippe, and J. L. Rosner, Phys. Rev. D 47, 1988-1993 (1993);
- M. Gronau and J. L. Rosner, Phys. Rev. Lett. 72, 195-198 (1994);
- Phys. Rev. D 49, 254-264 (1994);
- Phys. Rev. D 63, 054006 (2001).
- [60] OPAL Collaboration, K. Ackerstaff et al., Eur. Phys. J. C 5, 379-388 (()1998).
- [61] ALEPH Collaboration, R. Barate et al., Phys. Lett. B 492, 259-274 (2000).
- [62] The BaBar Physics Book: Physics at an Asymmetric B Factory, edited by P. F. Harrison and H. R. Quinn, SLAC Report SLAC-504, 1998.
- [63] M. Gronau, J. Rosner and D. London, Phys. Rev. Lett. 73, 21-24 (1994);
- M. Gronau and J. L. Rosner, Phys. Rev. Lett. 76, 1200-1203 (1996);
- Phys. Rev. D 57, 6843-6850 (1998);
- A. S. Dighe, M. Gronau, and J. L. Rosner, Phys. Rev. D 54, 3309-3320 (1996);
- A. S. Dighe and J. L. Rosner, 54, 4677-4679 (1996); M. Gronau and D. Pirjol, Phys. Lett. B 449, 321-327 (1999);
- Phys. Rev. D 61, 013005 (2000).
- [64] R. Fleischer, Phys. Lett. B 365, 399-406 (1996);
- Phys. Rev. D 58, 093001 (1998);
- R. Fleischer and T. Mannel, Phys. Rev. D 57, 2752-2759 (1998);
- A. J. Buras, R. Fleischer, and T. Mannel, Nucl. Phys. B533, 3-24 (1998);
- R. Fleischer and A. J. Buras, Eur. Phys. J. C 11, 93-109 (1999);
- A. J. Buras and R. Fleischer, Eur. Phys. J. C 16, 97-104 (2000).
- [65] M. Neubert and J. L. Rosner, Phys. Lett. B 441, 403-409 (1998);
- Phys. Rev. Lett. 81, 5076-5079 (1998);
- M. Neubert, JHEP 9902, 014 (1999).
- [66] N. G. Deshpande and X.-G. He, Phys. Rev. Lett. 74, 26-29 (1995); 74, 4099(E) (1995);
- O. F. Hernández, D. London, M. Gronau, and J. L. Rosner, Phys. Rev. D 52, 6374-6382 (1995).
- [67] G. Eilam, M. Gronau, and J. L. Rosner, Phys. Rev. D 39, 819-824 (1989).
- [68] M. Gronau and J. L. Rosner, Phys. Rev. D 61, 073008 (2000).
- [69] X.-G. He, W.-S. Hou, and K.-C. Yang, Phys. Rev. Lett. 83, 1100-1103 (1999);
- W.-S. Hou, J. G. Smith, and F. Würthwein, preprint hep-ex/9910014 (unpublished).
- [70] F. Würthwein and R. Jesik, talks for Working Group 1 presented at Workshop on B Physics at the Tevatron - Run II and Beyond, Fermilab, February 2000 (unpublished).
- [71] B. Blok, M. Gronau, and J. L. Rosner, Phys. Rev. Lett. 78, 3999-4002 (1997); 79, 1167 (1997);
- A. Falk, A. L. Kagan, Y. Nir, and A. A. Petrov, Phys. Rev. D 57, 4290-4300 (1998);
- M. Gronau and J. L. Rosner, Phys. Rev. D 57, 6843-6350 (1998); 58, 113005 (1998);
- R. Fleischer, Phys. Lett. B 435, 221-232 (1998);
- Eur. Phys. J. C 6, 451-470 (1999).
- [72] R. Fleischer, Phys. Lett. B 459, 306-320 (1999);
- Eur. Phys. J. C 16, 87-95 (2000).
- See also I. Dunietz, Proceedings of the Workshop on B Physics at Hadron Accelerators, Snowmass, CO, 1993, pp. 83-96;
- D. Pirjol, Phys. Rev. D 60, 054020 (1999).
- [73] M. Gronau and J. L. Rosner, Phys. Lett. B 482, 71-76 (2000).
- [74] M. Gronau, O. F. Hernández, D. London and J. L. Rosner, Phys. Rev. D 50, 4529-4543 (1994).
- [75] CLEO Collaboration, S. Chen et al., Phys. Rev. Lett. 85, 525-529 (2000).
- [76] M. Gronau and J. L. Rosner, Phys. Rev. D 59, 113002 (1999).
- [77] H. Georgi, Phys. Lett. B 297, 353-357 (1992);
- L. Wolfenstein, Phys. Lett. B 164, 170-172 (1985);
- Phys. Rev. Lett. 75, 2460-2461 (1995);
- J. Donoghue, E. Golowich, B. R. Holstein, and J. Trampeti\'c, Phys. Rev. D 33, 179-183 (1986).
- [78] CLEO Collaboration, R. Godang et al., Phys. Rev. Lett. 84, 5038-5042 (2000).
- [79] FOCUS Collaboration, J. M. Link, et al., Phys. Rev. B 485, 62-70 (2000);
- Phys. Rev. Lett. 86, 2955-2958 (2001).
- [80] Fermilab E791 Collaboration, presented by A. J. Schwartz, Univ. of Cincinnati report UCTP-112-00, hep-ex/0012006 (unpublished);
- J. A. Appel, hep-ex/0002022, in Proceedings of the 3rd Workshop on Physics and Detectors for DAPHNE [25], pp. 231-247.
- [81] A. F. Falk, Y. Nir, and A. A. Petrov, JHEP 12, 019 (1999);
- S. Bergmann, Y. Grossman, Z. Ligeti, Y. Nir and A. A. Petrov, Phys. Lett. B 486, 418-425 (2000);
- I. I. Bigi and N. G. Uraltsev, Nucl. Phys. B592, 92-106 (2000).
- [82] See, e.g., FOCUS Collaboration, J. M. Link et al., Phys. Lett. B 491, 232-239 (2000); 495, 443(E) (2000).
- [83] CLEO Collaboration, D. Cronin-Hennessy et al., Phys. Rev. D 86, 3730-3734 (2001).
- [84] CLEO Collaboration, M. Artuso et al., Cornell University report CLNS 00-1698, hep-ex/0010080.
- [85] CLEO Collaboration, S. E. Csorna et al., Phys. Rev. 86, 4243-4246 (2001).
- [86] CLEO Collaboration, S. Anderson et al., Nucl. Phys. A663, 647-650 (2000).
- [87] FOCUS Collaboration, presented by F. L. Fabbri at Osaka Conf. [26], hep-ex/0011044;
- presented by C. Ricciardi, Nucl. Phys. A663, 651-654 (2000).
- [88] KOPIO Collaboration, in Rare Symmetry Violating Processes, proposal to the National Science Foundation, October 1999, and Brookhaven National Laboratory Proposal P926 (unpublished).
- [89] KAMI Collaboration, Fermilab Proposal P804 (unpublished).
- [90] CKM Collaboration, Fermilab Proposal P905.
- [91] SNO Collaboration, presented by R. van de Water in Particle Physics and Cosmology: 2nd Tropical Workshop [1], pp. 193-200.
- [93] Super-Kamiokande Collaboration, presented by M. Vagins in Particle Physics and Cosmology: 2nd Tropical Workshop [1], pp. 122-192.
- [94] NuTeV Collaboration, presented by J. Conrad in Particle Physics and Cosmology: 2nd Tropical Workshop [1], pp. 331-340;
- [95] J. L. Rosner, Nucl. Phys. B Proc. Suppl. 73, 29-42 (1999).
- [96] P. Burchat et al., Report of the NSF Elementary Particle Physics Special Emphasis Panel on B Physics, July, 1998 (unpublished).
- [97] See, e.g., C. O. Dib, D. London, and Y. Nir, Int. J. Mod. Phys. A 6, 1253-1266 (1991);
- M. Gronau and D. London, Phys. Rev. D 55, 2845-2861 (1997).
- [98] Y. Grossman and M. P. Worah, Phys. Lett. B 395, 241-249 (1997);
- Y. Grossman, G. Isidori, and M. P. Worah, Phys. Rev. D 58, 057504 (1998).
- [99] A. D. Sakharov, Pis'ma Zh. Eksp. Teor. Fiz. 5, 32-35 (1967)
- [JETP Lett. 5, 24-27 (1967)].
- [100] M. P. Worah, Phys. Rev. D 56, 2010-2018 (1997);
- Phys. Rev. Lett. 79, 3810-3813 (1997);
- M. Carena, M. Quiros, A. Riotto, I. Vilja, and C. E. M. Wagner, Nucl. Phys. B503, 387-404 (1997);
- [101] P. Ramond, in Particle Physics and Cosmology: 2nd Tropical Workshop [1], pp. 75-90.
- [102] G. 't Hooft, Phys. Rev. Lett. 37, 8-11 (1976);
- M. Fukugita and T. Yanagida, Phys. Lett. B 174, 45-47 (1986);
- M. A. Luty, Phys. Rev. D 45, 455-465 (1992);
- M. Plümacher, Zeit. Phys. C 74, 549-559 (1997);
- W. Buchmüller and M. Plumacher, Phys. Lett. B 389, 73-77 (1996); 431, 354-362 (1998);
- Phys. Rep. 320, 329-339 (1999).
- [103] J. L. Rosner, in The Albuquerque Meeting (Proceedings of the 8th Meeting, Division of Particles and Fields of the American Physical Society, Aug. 2-6, 1994, The University of New Mexico), edited by S. Seidel (World Scientific, Singapore, 1995), pp. 321-350;
- M. P. Worah, Phys. Rev. D 53, 3902-3912 (1996).
Publication Dates
-
Publication in this collection
13 Mar 2002 -
Date of issue
June 2001
History
-
Received
03 Jan 2001