Abstract
Improving adsorptive processes demands a constant search for new adsorbents. In the specific case of ethanol-water separation, A zeolites are successfully being used. The use of nonconventional adsorbents to substitute zeolites, mainly starchy adsorbents in virtue of their known chemical affinity water, has recently been proposed. In this work a thermodynamic and kinetic study has been undertaken on the liquid phase adsorption of water from an ethanol-water mixture using manioc starch pellets as the adsorbent. The fundamental thermodynamic data were obtained by means of the static method, using a thermostated bath at four different temperatures (25, 40, 50 and 60° C), and could be correlated by means of a semi-empirical isotherm. The kinetic data, in turn, were obtained in a finite circulating liquid bath cell, enabling the construction of uptake rate curves, whereby the influence of temperature, interstitial velocity and adsorbent mean particle size on the adsorption rate was analyzed. The effective internal diffusivities at the experimental temperatures were estimated by a pore diffusion model and the results obtained were compared with those for commercial 3A zeolite
Ethanol; starch; adsorption
KINETIC AND THERMODYNAMIC STUDY ON ADSORPTION BY STARCHY MATERIALS IN THE ETHANOL-WATER SYSTEM
M.J. Carmo and J.C. Gubulin
Universidade Federal de São Carlos - Departamento de Engenharia Química - Rod. Washington Luiz, Km 235
CEP: 13565-905, São Carlos - SP - Brazil - Phone: (016) 274-8264; Fax: (016) 274-8266
(Received: March 5, 1997; Accepted: August 5, 1997)
Abstract: Improving adsorptive processes demands a constant search for new adsorbents. In the specific case of ethanol-water separation, A zeolites are successfully being used. The use of nonconventional adsorbents to substitute zeolites, mainly starchy adsorbents in virtue of their known chemical affinity water, has recently been proposed. In this work a thermodynamic and kinetic study has been undertaken on the liquid phase adsorption of water from an ethanol-water mixture using manioc starch pellets as the adsorbent. The fundamental thermodynamic data were obtained by means of the static method, using a thermostated bath at four different temperatures (25, 40, 50 and 60° C), and could be correlated by means of a semi-empirical isotherm. The kinetic data, in turn, were obtained in a finite circulating liquid bath cell, enabling the construction of uptake rate curves, whereby the influence of temperature, interstitial velocity and adsorbent mean particle size on the adsorption rate was analyzed. The effective internal diffusivities at the experimental temperatures were estimated by a pore diffusion model and the results obtained were compared with those for commercial 3A zeolite.
Keywords: Ethanol, starch, adsorption
INTRODUCTION
One of the main problems related to the production of anhydride ethanol derived from fermentation is the high energy cost of separating ethanol from the fermenting must. When distillation is used to dehydrate ethanol, 50% of the total energy is consumed. This frequently results in a negative energy balance, where the energy spent on the anhydride ethanol production exceeds the energy obtained from its combustion.
An alternative to this process is the use of preferential adsorption of water, using specific adsorbents for this purpose, such as cellulose and starch, first studied by Ladisch and Dick (1979).
Since then, numerous studies have proven that it is possible to use biomaterials for the dehydration of ethanol (Hong et al., 1982; Robertson et al., 1983; Hills and Pirzada, 1989 and Crawshaw and Hills, 1990). The advantages of their use include efficiency, relatively low cost, reuse of the material in fermentation and/or animal feedstock, in addition to the fact that these adsorbents are nontoxic, biodegradable and derived from renewable sources.
In this work a study was carried out in order to obtain fundamental thermodynamic and kinetic liquid phase adsorption data for the ethanol-water/starch system with the aim to define the capacities and diffusivities at four different operational temperatures.
MATERIALS AND METHODS
Spherical manioc starch pellets, commercially obtained in 500g packages with diameters ranging from 2.00 to 4.00 mm, were used. Physical characterization of this material included mean diameter obtained by the Tyler/Mesh procedure, real density, apparent density and porosity by means of water picnometry. The results are listed in Table 1.
The ethanol-water solutions were prepared at the required mass concentrations (concentration range of from 0 to 50 wt% ethanol for the thermodynamic tests and around 90 wt% ethanol for the kinetic tests) from ethanol of the Synth brand and distilled water, using a scale with an accuracy of 0.001g. The comercial ethanol was treated with previously activated 3A zeolite (300° C for 24 h) in order to remove any remaining water.
For measuring the concentration of the fluid phase, a Reichert-Jung Auto Abbé refractometer with automatic calibration was used and data reproducibility on the order of 0.5% was obtained in this experimental concentration range.
EXPERIMENTAL PROCEDURE
The equilibrium data were obtained by the static method, which consists in placing inside hermetic 125 ml flasks a specific amount Ms of thermally treated (105° C for 16 h) adsorbent (» 15g) in contact with a specific mass Mf of aqueous ethanol solution (» 40g) of a well-defined initial concentration . Several initial concentrations were used in order to obtain a wide range of isotherms at four different temperatures (25, 40, 50 and 60° C). The flasks were maintained in a thermostated bath with an accuracy of ± 0.1° C, and gently shaken during approximately 7 days, after which the fluid phase concentration was measured and the end concentration
of the liquid was determined.
In order to obtain the amount of water adsorbed in the solid phase, a mass balance was used between the phases, where ethanol was considered the non adsorbable component. This hypothesis agrees with the work of Rebar et al. (1984), where it was demonstrated that the chemical affinity of starch to water is much greater
than that of ethanol. The mass balance is described by equation 1, where at equilibrium when t® ¥ ,
(1)
To obtain the kinetic data Azevedo (1992) developed an experimental circulating device (fig. 1) within which a finite volume of liquid (» 250 ml) circulates continuously in a closed loop and through a packed bed of adsorbent particles (» 50g).
This device permits flow conditions at which the external resistances to mass transfer become negligible. The equipment possesses a centrifugal pump that continuously removes liquid at the bottom of the cell and sends this liquid to the top in a closed loop. At regular time intervals the liquid was measured. The concentration of the adsorbed phase was obtained by a simple mass balance, which considered ethanol as the nonadsorbable component, according to equation 1.
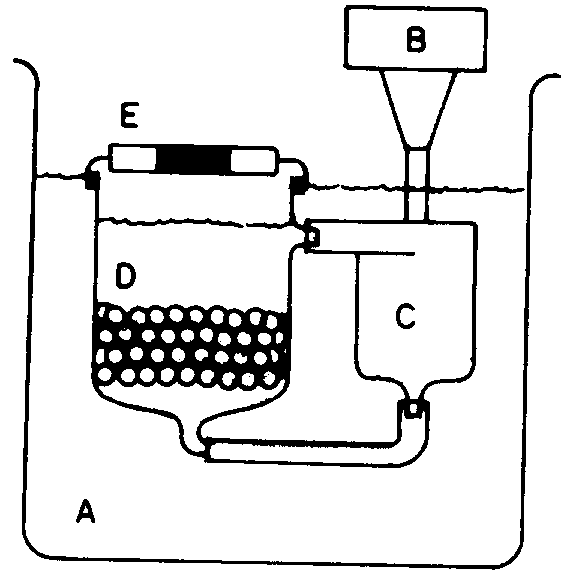
B -Variable Motor Rotation
C -Centrifugal Pump
D - Cell (particles and fluid)
E - Lid (with septum )
For a typical kinetic mass transfer test, ethanol-water solutions were prepared with mass Mf (» 200g) and initial concentration (» 10 wt% water) and placed in a hermetic thermostated cell. It was certified that the liquid filled the pump completely without the formation of bubbles. An acrylic support was used to accommodate the cell-pump setup in the thermostated bath, attaching the pump shaft to the variable rotation motor starting the liquid circulation.
As soon as the system attained thermal equilibrium, a device in the lid was removed from the cell and at time t=0 a mass of thermally treated adsorbent Ms was introduced into the cell as quickly as possible. The system was then hermetically closed and at regular time intervals liquid samples were taken by puncturing a rubber septum with a long hypodermic needle. The sample concentration were measured by means of refractometry, with a reproducibility of 0.5% in the experimental concentration range.
RESULTS AND DISCUSSION
Equilibrium Data
The isotherms obtained, relate which the concentrations in the liquid and solid phases at the temperatures of 25, 40, 50 and 60° C, can be seen in Figure 2.
From the isotherms one may note that there is a decrease in the adsorbing capacity (represented by the horizontal plateau) as the temperature increases. This is because of the increase in the vibrational energy of the molecules, which at higher temperatures allows a smaller net number of molecules to be adsorbed at equilibrium, since due to the exothermic character of the adsorption process, an increase in temperature shifts the equilibrium towards the region unfavorable to adsorption. A semi-empirical model was used which satisfactorily correlated the equilibrium data in this temperature range. The model is represented by the Chakravarti-Dhar (1907) isotherm, defined by equation 2.
(2)
The parameters of this equation could be related to the temperature via a computer program, by using an estimation of nonlinear parameters. Table 2 shows the results obtained.
Figure 2: Adsorption isotherms for the ethanol-water/starch system.
From these relations the parameters could be equated by polynomials as a function of temperature within the range of 298-333K. The equations are presented as follows:
(3)
(4)
u = - 10.846 + 0.0393T (5)
Kinetic Data
The kinetic results are presented by means of uptake rate curves, relating the amount of water adsorbed by the solid (q) or standardizing this quantity by means of the dimensionless concentration in the adsorbed phase (y ) for each time. Parameters like interstitial velocity, mean particle diameter and temperature were related to the adsorption rates. The pore diffusion model was applied, with the diffusion resistance concentrated in the macropores, which satisfactorily represented the experimental data, making it possible to estimate the diffusivities for several temperatures and particle diameters.
Analysis of the effect of the interstitial velocity on the mass transfer rate showed that as this velocity is increased more water is adsorbed. This happens due to a decrease in the thickness of the hydrodynamic boundary layer surrounding the particle, where external mass transfer resistance takes place. Figure 3 illustrates this, demonstrating that an increase in interstitial velocity results in an increase in adsorption rates.
Figure 3: Kinetic curves for several interstitial velocities - Dp = 2.19mm , q =25° C.
Figure 4: Uptake rate curves for several particle sizes - VI > 4 cm/s and T = 25° C.
The pellet size also affects the adsorbents uptake rates. From the results obtained and plotted in Figure 4, one may note that the adsorption rates increase as the mean particle size of the adsorbent decreases. This may be explained by the larger total area of agglomerates of smaller particles in relation to the same mass of larger particles, decreasing the difusional path of the water molecules. This results in a greater period of time spent to hydrate all the hydroxyl amide groups.
The temperature is another feature of great interest in kinetic adsorption processes, as its influence on the diffusion process is very significant. From Figure 5 one may note that as temperature increases the adsorption rate also increases. At the interval of 60 minutes, the adsorbent attained approximately 90% saturation at 60° C, while at the same interval the adsorbent attained around 70% at 25° C. This occurs due to an increase in the degree of molecular agitation, facilitating the adsorption kinetics.
The kinetic data for the starch were compared to those for a conventional adsorbent, such as commercial 3A zeolite, obtained by Azevedo (1993) under the same operational conditions. Figure 6 shows the saturation trend for these two adsorbents at 25° C, where for long periods of time the zeolite tends to become saturated at 30% of its dry mass, while for starch this saturation tends to be at 15%. In comparing the adsorption rates, Figure 7 shows that the zeolite presents a higher uptake rate in relation to that of the starch, as at all times its dimensionless concentration in the adsorbed phase was superior.
Figure 5: Uptake rate curves for several temperatures - Dp = 2.19mm and VI>4 cm/s.
Figure 6: Plot comparing adsorption capacity between starch and zeolite.
Figure 7: Uptake rates for the starchy and zeolite adsorbents.
Table 3 shows the values obtained for the diffusivities in several kinetic experiments using the pore diffusion model.
The results obtained showed that the mean particle size does not significantly affect the diffusivity values, since an increase in the adsorption rate is compensated by the decrease in surface area of the smaller size particles. The temperature, in contrast, had a pronounced effect on the diffusivity values, favoring the kinetics process in virtue of an increase in molecular agitation.
CONCLUSIONS
The thermodynamic results confirm the affinity of starch to water in the presence of ethanol. In terms of adsorption capacity, manioc starch adsorbs water at a proportion of 15% of its dry mass at 25° C. This capacity decreases as temperature increases. In terms of a thermodynamic model representing the data in a satisfactory way, the Chakravarti isotherm (1907) was shown to be appropriate for the temperature range employed. The equipment used and the methodology employed were shown to be satisfactory in obtaining the experimental kinetic adsorption data for this unconventional adsorbent. The kinetic adsorption process was dependent on the parameters studied: temperature, interstitial velocity and particle diameter. It was concluded that a temperature increase provokes an increase in the adsorption rate, but it decreases the capacity to retain water during long periods of time. The increase in interstitial velocity decreases the external resistance to mass transfer to a limit at which the liquid film surrounding the particle becomes extremely thin. An increase in the mean particle diameter of the starchy material causes a decrease in the adsorption rates. Compared to 3A zeolite, starch showed a smaller capacity and lower adsorption rates for the entire temperature range studied.
NOMENCLATURE
c* Concentration of the liquid phase at equilibrium, weight % water
D Effective diffusivity, cm2/s
Dp Diameter of particle, cm
Km Chakravartis constant, ml/g
Mf Mass of liquid, g
Ms Mass of adsorbent, g
q* Concentration of the adsorbed phase at equilibrium, g/gads
qs Concentration of the adsorbed phase after a specific time interval, g/gads
q0 Capacity of the mono layer, g/gads
T Temperature, K
VI Interstitial velocity, cm/s
Mass fraction of ethanol in the solution at equilibrium, weight % ethanol
Initial mass fraction of ethanol in the solution, weight % ethanol
Wb Dass fraction of ethanol in the solution after a specific time interval, weight % ethanol
u Chakravartis constant
y Dimensionless concentration in adsorbed phase
REFERENCES
Azevedo, D.C.S., Estudo Cinético e Termodinâmico de Adsorção para o Sistema Etanol-Água sobre Zeólita 3A, Ph.D. diss., Universidade Federal de São Carlos, 195 (1992).
Carmo, M.J., Estudo Cinético e Termodinâmico da Adsorção para o Sistema Etanol- Água sobre Matéria Prima Amilácea, Ph.D. diss., Universidade Federal de São Carlos, 108 (1995).
Crawshaw, J.P and Hills, J.H., Sorption of Ethanol and Water by Starchy Materials, Ind. Eng. Chem. Res, 29, 307 (1990).
Chakravarti, D.N. and Dhar, N.R., Kolloid Z, 43, 377 (1907).
Hong, J., Voloch, M., Ladisch, M.R. and Tsao, G.T., Adsorption of Ethanol-Water Mixtures by Biomass Materials, Biotechnol. Bioeng, 24, 725 (1982).
Hills, J.H. and Pirzada, I.M., Analysis and Prediction of Breakthrough Curves for Packed Bed Adsorption of Water Vapour on Corn-Meal, Chem. Eng. Res. Des, 67 (1989).
Ladisch, M.R. and Dick, K., Dehydration of Ethanol: New Approach Gives Positive Energy Balance, Science, 24, 725 (1979).
Robertson, G.H., Doyle, L.R., and Pavlath, A.E., Intensive Use of Biomass Feedstock in Ethanol Conversion: The Alcohol-Water Vapor Phase Separation, Biotechnol. Bioeng, 25, 3133 (1983).
Rebar, V., Fischbach, E.R., Apostopoulos, D. and Kokini, J.L., Thermodynamics of Water and Ethanol Adsorption on Four Starches as Model Biomass Separation Systems, Biotechnol. Bioeng, 25, 3133 (1984).
Publication Dates
-
Publication in this collection
09 Oct 1998 -
Date of issue
Sept 1997