Abstracts
In order to evaluate the response of Beijerinckia derxii ICB-10 to different environmental factors, growth curves and specific nitrogenase activity were studied. Tested conditions were as follows: a) media with different pH values (2.5, 2.8, 4.2 and 5.7); b) medium supplemented with 230 µM aluminium sulphate; c) media with two different potassium phosphate concentrations (50 mM and 100 mM); d) shaken or still cultures; e) medium supplemented with 40 mM sodium thiosulphate. Growth curves and specific nitrogenase activity at pH 4.2 were closely similar to those for cultures at pH 5.7 (standard condition), whereas no growth occurred at pH 2.5. Changes in growth curves and/or specific nitrogenase activity were observed under the following conditions: I) pH 2.8 (decrease in initial CFU number, reduction of maximum specific growth rate, reduced number of generations and stimulation of nitrogenase activity), II) presence of aluminium (early death phase), III) 50 mM PO4(3-) (reduction of maximum specific growth rate), IV) 100 mM PO4(3-) (reduction of both number of generations and maximum specific growth rate as well as early death phase), V) low O2 availability (increasing nitrogenase activity), and VI) presence of thiosulphate (reduction of maximum specific growth rate; early death phase and high stimulation of nitrogenase activity). The data obtained showed the high variability of the cell growth response to environmental factors. Nitrogenase activity was always preserved even when population growth was affected.
Beijerinckia; nitrogenase; nitrogen fixation; growth; environmental factors
Os parâmetros utilizados para se avaliar a ação de diferentes fatores ambientais sobre Beijerinckia derxii foram curvas de crescimento e medidas de atividade específica de nitrogenase. As condições estudadas foram: a) meios com os valores de pH 2,5, 2,8, 4,2 e 5,7; b) meio suplementado com 230 mM de sulfato de alumínio; c) meio com as concentrações de fosfato de potássio: 50 mM and 100 mM; d) culturas agitada e estacionada; e) meio suplementado com 40 mM de tiossulfato de sódio. O crescimento e a atividade da nitrogenase foram muito similares em meio com pH 4,2 e 5,7 (condição padrão). Em pH 2,5 não se observou crescimento. As seguintes condições provocaram alterações no perfil da curva de crescimento ou na atividade da enzima ou ambos: pH 2,8 (diminuição do número inicial de UFC, redução da máxima velocidade especifica de crescimento, redução no número de gerações e estímulo na atividade da nitrogenase), presença de alumínio (fase de declínio precoce), 50 mM PO4(3-) (redução da velocidade especifica de crescimento máxima), 100 mM PO4(3-) (redução da velocidade especifica de crescimento máxima e no número de gerações e fase de declínio precoce), baixa disponibilidade de O2 (estímulo na atividade da nitrogenase) e presença de tiossulfato (redução da velocidade especifica de crescimento máxima, elevado estímulo na atividade da nitrogenase e fase de declínio precoce). Ocorreu uma variação no perfil das curvas de crescimento em resposta à ação dos fatores ambientais. A atividade da nitrogenase foi sempre mantida, mesmo quando o crescimento foi reduzido.
Beijerinckia; nitrogenase; fixação de nitrogênio; crescimento; fatores ambientais
Nitrogenase activity of Beijerinckia derxii is preserved under adverse conditions for its growth
A atividade da nitrogenase de Beijerinckia derxii é preservada sob condições diversas para seu crescimento
Heloiza R. BarbosaI;Marcos A. MorettiI; Daniela S. ThulerI; Elisabeth F.P. AugustoII
IIIGrupo de Biotecnologia, Departamento de Química, Instituto de Pesquisa e Tecnologia IPT, São Paulo, SP, Brasil.
CorrespondenceCorrespondence to Departamento de Microbiologia Instituto de Ciências Biomédicas Universidade de São Paulo Av. Prof. Lineu Prestes, 1374, Cidade Universitária, 05508-900, São Paulo, SP, Brasil Fax: (+5511) 3818-7354 E-mail: hrbarbos@.icb.usp.br
ABSTRACT
In order to evaluate the response of Beijerinckia derxii ICB-10 to different environmental factors, growth curves and specific nitrogenase activity were studied. Tested conditions were as follows: a) media with different pH values (2.5, 2.8, 4.2 and 5.7); b) medium supplemented with 230 µM aluminium sulphate; c) media with two different potassium phosphate concentrations (50 mM and 100 mM); d) shaken or still cultures; e) medium supplemented with 40 mM sodium thiosulphate. Growth curves and specific nitrogenase activity at pH 4.2 were closely similar to those for cultures at pH 5.7 (standard condition), whereas no growth occurred at pH 2.5. Changes in growth curves and/or specific nitrogenase activity were observed under the following conditions: I) pH 2.8 (decrease in initial CFU number, reduction of maximum specific growth rate, reduced number of generations and stimulation of nitrogenase activity), II) presence of aluminium (early death phase), III) 50 mM PO43- (reduction of maximum specific growth rate), IV) 100 mM PO43- (reduction of both number of generations and maximum specific growth rate as well as early death phase), V) low O2 availability (increasing nitrogenase activity), and VI) presence of thiosulphate (reduction of maximum specific growth rate; early death phase and high stimulation of nitrogenase activity). The data obtained showed the high variability of the cell growth response to environmental factors. Nitrogenase activity was always preserved even when population growth was affected.
Key words: Beijerinckia, nitrogenase, nitrogen fixation, growth, environmental factors
RESUMO
Os parâmetros utilizados para se avaliar a ação de diferentes fatores ambientais sobre Beijerinckia derxii foram curvas de crescimento e medidas de atividade específica de nitrogenase. As condições estudadas foram: a) meios com os valores de pH 2,5, 2,8, 4,2 e 5,7; b) meio suplementado com 230 mM de sulfato de alumínio; c) meio com as concentrações de fosfato de potássio: 50 mM and 100 mM; d) culturas agitada e estacionada; e) meio suplementado com 40 mM de tiossulfato de sódio. O crescimento e a atividade da nitrogenase foram muito similares em meio com pH 4,2 e 5,7 (condição padrão). Em pH 2,5 não se observou crescimento. As seguintes condições provocaram alterações no perfil da curva de crescimento ou na atividade da enzima ou ambos: pH 2,8 (diminuição do número inicial de UFC, redução da máxima velocidade especifica de crescimento, redução no número de gerações e estímulo na atividade da nitrogenase), presença de alumínio (fase de declínio precoce), 50 mM PO43- (redução da velocidade especifica de crescimento máxima), 100 mM PO43- (redução da velocidade especifica de crescimento máxima e no número de gerações e fase de declínio precoce), baixa disponibilidade de O2 (estímulo na atividade da nitrogenase) e presença de tiossulfato (redução da velocidade especifica de crescimento máxima, elevado estímulo na atividade da nitrogenase e fase de declínio precoce). Ocorreu uma variação no perfil das curvas de crescimento em resposta à ação dos fatores ambientais. A atividade da nitrogenase foi sempre mantida, mesmo quando o crescimento foi reduzido.
Palavras-chave: Beijerinckia, nitrogenase, fixação de nitrogênio, crescimento, fatores ambientais
INTRODUCTION
The importance of the nitrogen cycle in productivity of the biosphere has caused many authors to focus their attention on nitrogen fixation, which is its rate-determining step of the N cycle (18). Consequently, considerable research effort has been devoted to understand and overcoming inefficiencies in this process.
Environmental factors may repress nitrogen-fixing bacteria. They may act on physiological properties restricting growth or inhibiting the nitrogenase activity, thus, lowering the contribution of these microorganisms to the environment. Several authors have studied the effects of chemical factors both on axenic cultures or on the environment. (12,14,19,21).
Free-living nitrogen-fixing bacteria from the genus Beijerinckia are commonly isolated from lateritic tropical soils. Laterization leads to accumulation of aluminium, iron, titanium and manganese, while there is simultaneously a leaching away of bases such as calcium, potassium, magnesium and sodium. The low pHs values, generally found in these soils, are responsible for low phosphate and nitrogen availability. (7). Considering these characteristics, Becking (8) showed that pH 3.0 is the lowest value in which the genus Beijerinckia is able to grow and fix nitrogen; but he did not determine the effect of pH on growth in quantitative terms. The same author also showed that Al3+, PO43- and other minerals (Ca2+, K+, Fe2+, Fe3+ etc) may function as nutrients or inhibitors of Beijerinckia and Azotobacter growth. The actual effect of these minerals depends on the type of element, its concentration, the pH of the medium in which the effect of those elements was tested and the microorganism itself; since nitrogenase activity was determined only indirectly on the basis of the nitrogen incorporated by bacterial cells, the relation between this enzyme and cell physiology was not investigated.
Oxygen is one of the most important factors interfering with nitrogenase activity. Due to nitrogenase O2-lability, N2-fixing organisms require low oxygen pressures or protective mechanisms to maintain the enzyme activity (18). Excessive O2 inhibits nitrogenase activity, but lack of this element results in a reduction of bacterial growth (11). In Beijerinckia derxii strain ICB-10 the mucous layer functions as a morphological protective mechanism that allows nitrogenase activity under high agitation (4). However, there are no data concerning the enzyme activity under low conditions of oxygen availability.
The association of a Beijerinckia with the leaching bacterium Thiobacillus ferrooxidans was found to enhance the rate and the extent of copper and nickel ore leaching, demonstrating that nitrogen fixation can be important in bioextractive metallurgy (22,24). However, there is no data on the interference of elements such as sulphur compounds, not commonly occurring in soil, but present in leaching processes, on the physiology of Beijerinckia.
Whatever the potential applications of Beijerinckia may be, very little information is available about the adverse effect of intrinsic soil characteristics (lack of nutrients, excessive amounts of inhibitors, etc.) and leaching conditions (low pH and high mineral concentrations) on the bacterial physiology.
The aim of the present study was to verify the possible effects of some environmental factors on the growth and nitrogenase activity of Beijerinckia derxii strain ICB-10. Nitrogenase activity profiles in cultures grown at low pH values (2.8 and 4.2), with different phosphate concentrations, in the presence of aluminium and S2O32- and low O2 availability were drawn to establish a correlation between nitrogenase activity profiles and growth curves obtained under the same growth conditions.
MATERIALS AND METHODS
Microorganism
The microorganism, identified as Beijerinckia derxii - ICB-10 (ATCC 33962) (2) was isolated from latosol-red yellow soil under shrubland cover in Pirassununga, São Paulo, Brazil. Some chemical characteristics of the soil were: pH 4.2, 0.77% organic matter, scarcity of phosphates and high aluminium concentrations that predominantly ranged from 6.9 to 14.4 mg per 100 g of soil, although lower levels may also be present (15).
Culture media and conditions
Basic medium (medium B) consisted of (g.L-1): K2HPO4 - 0.10; CaCl2.2H2O- 0.02; MgS04.7H2O - 0.2; Na2MoO4.2H2O - 0.002; KH2PO4 - 0.30; FeCl3.6H2O- 0.01; CoCl2.H2O - 0.0008 and glucose - 10; pH 5.7. For solid medium, 12 g.L-1 of agar were added to liquid basic medium.
The experimental conditions were as follows: condition A was the standard one, where basic medium was used to grow shaken cultures at 200 rpm in a model G-25 rotary shaker "New Brunswick Sci Co", N.J., U.S.A. The other conditions were modifications of condition A. In condition B, C and D the pH of the media were 2.5, 2.8 and 4.2, respectively. In condition E, 230 µM Al2(SO4)3 was added and pH was 4.2. In conditions F and G the phosphate concentrations were 50 mM and 100 mM, respectively. In condition H, the culture was not agitated. In condition I, 40 mM Na2S2O3 was added. The pH of the media was adjusted with 0.1M HCl in a model H-5 pHmeter, "Horiba" Kyoto, Japan, before sterilization (121ºC, 20 min) and checked afterwards. In conditions F, G, H and I the pH was 5.7. The Al2(SO4)3 concentration was the same as employed by Becking (8) including the eventual contaminants in the standard medium.
Sampling
A 72 h culture grown on B medium was used as inoculum at 5% (v/v) concentration. Cultures were grown in 500 mL conical flasks containing 100 mL of medium and incubated at 30ºC. Samples were taken periodically for evaluation of colony forming units (CFU) by the drop method (5) and of nitrogenase activity by the acetylene reduction method (23). In the nitrogenase activity assays, 1.0 mL of the culture was added to a tube with a rubber stopper and 10% of the gas phase was withdrawn with a syringe and replaced with acetylene. The flasks were incubated for 2 h at 30ºC in a model R-25 reciprocating shaker "New Brunswick Sci Co", N.J. U.S.A. Ethylene (C2H4) was measured using a model GC-14A gas chromatograph "Shimadzu", Kyoto, Japan equipped with stainless steel columns packed with Porapak N and a hydrogen flame ionization detector. The nitrogenase activity was expressed as the mean of three determinations. Using the area values obtained from the chromatogram, specific nitrogenase activity (A) was calculated according to the expression:

Vf = Flask volume (L);
Va = sample volume (L);
t = reaction time (h);
CFU = colony forming units.mL-1;
nA, the number of mols of each sample, was obtained as follows;
nA= nB. sample area / C2H4 standard area;
C2H4 standard area was a mean of three chromatographic determinations;
nB= n / standard flask volume;
n, the number of mols of C2H4 standard, was calculated using the expression n = P V/ R T;
P= pressure in atm;
V= volume (L) of C2H4 used to prepare the standard flask;
R= the gas constant, 0.082 atm.L.K-1.mol-1;
T= temperature in ºK.
Linear regression fittings (least square method) were applied to ln (CFU number) curves in order to obtain maximum growth rate values (µmax) for each condition (17). The same methodology was used to determine the specific death rate (KD) during the death phase.
The Student t-test (a = 0.05) was applied in order to determine significant differences between the mean µmax value of each environmental condition tested and the standard condition (condition A). The number of generations (n) was calculated according to the expression:
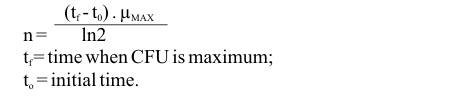
tf = time when CFU is maximum;
to = initial time.
RESULTS
Influence of pH
Fig. 1 and Table 1 show the growth and specific nitrogenase activity profiles of B. derxii strain ICB-10 under conditions A, C, and D (see Material and Methods). At pH 5.7 (condition A) the microorganism presented the following characteristics: µmax= 0.0592 h-1, a stationary phase observed from 70 to 260 hours, no death phase, and n = 5.1. The specific nitrogenase activity, restricted to the exponential growth phase, showed a single peak with a maximum value of about 2.0 fmol.h-1.CFU-1 C2H4. No growth occurred at pH 2.5 (condition B). The characteristic pattern of the growth curve at pH 2.8 (condition C) showed a decline of the initial CFU number up to 80 hours followed by an increase in the remaining population, reaching the initial CFU number at a µmax = 0.0198 h-1 and n = 3.5; the specific nitrogenase activity increased at the beginning of culture, presenting a higher peak than the standard condition during the exponential phase. The growth and specific nitrogenase activity curves at pH 4.2 (condition D) were very similar to those obtained for condition A; the difference was a higher n value (n = 6). During the development of the populations, the pH values of the media did not suffer considerable alterations (about 0.1 pH unity).Influence of aluminium
Fig. 2 and Table 1 show that culture growth was partially affected in condition E. The µmax and n values were not affected compared to condition D. However, a decline in the number of CFU, with a KD= -0.0222 h-1, was observed immediately after the end of the exponential phase. Specific nitrogenase activity was not affected in comparison to condition D.Influence of phosphate concentration
Changes in µmax values, were proportional to the concentration of phosphate (conditions F and G) as shown in
Fig. 3 and Table 1. Populations grown in condition F showed a µmax = 0.0375 h-1, an n value (4.7) close to the standard values and a stable stationary phase up to 180 h, after which they underwent a subtle decline with KD = -0.0034 h-1. On the other hand, populations grown in condition G showed a µmax = 0.0268 h-1, reached an n value = 2.1 and started to decline immediately after the exponential phase with KD = -0.0088 h-1. The specific nitrogenase activity for the two tested phosphate concentrations showed very similar profiles which were also similar to the standard profile (Fig. 1).Influence of O2 availability
Compared to the shaken culture, the unshaken culture (condition H) presented a practically constant cell number throughout the assay (
Fig. 4), although a gradual increase in specific nitrogenase activity was observed; the enzyme activity was still high at the end of the test, being comparable to the peaks observed for the shaken culture.Influence of sodium thiosulphate
As indicated by the low µmax value (0.0227 h-1), the low n (2.4) and the establishment of the decline phase after 90 h (KD = - 0.0294 h-1) (Table 1), bacterial growth (
Fig. 5) was quite affected by the presence of thiosulphate (condition I). The peak of specific nitrogenase activity in the exponential phase was about 9 times higher than that observed under standard conditions. Nevertheless, another peak with a much higher value was observed during the death phase.Statistically significant differences in mmax values compared to the standard condition were observed only for the cultures at pH 2.8, at 50 mM and 100 mM phosphate concentrations and in the presence of thiosulphate (Table 1).
DISCUSSION
The growth and specific nitrogenase activity curves of B. derxii strain ICB-10 permitted us to determine both the stimulation and inhibition by each test-agent and also at what growth phase the effect occurred.
The lowest pH value under which B. derxii strain ICB-10 was able to grow (pH 2.8) was very similar to that observed by Becking (7). The results shown in Fig. 1 suggest that part of the population was unable to survive at this pH but that the remaining cells were still able to perform some division. Despite the low µmax (0.0198 h-1) and n (3.5), these cells showed a stimulus of the nitrogenase activity, presenting a higher peak than the standard one during the exponential phase in condition A.
The ability to multiply at low pH depends on accurate responses that control the passage of protons into the cell. Pham and Burgess (16), working with purified nitrogenase from Azotobacter vinelandii, demonstrated that the enzyme was inactivated at pH lower than 5.0. The fact that the nitrogenase enzyme is active when the bacterial cell is in an acid environment means that propitious cytoplasmic conditions are being maintained. In order to increase the chance of surviving under the stress conditions imposed by a low pH, the microorganisms undergo a programmed molecular response by which specific, stress-inducible proteins are synthesized. These proteins presumably act to prevent or repair the macromolecular damages caused by stress (6). The growth and specific nitrogenase activity curves at pH 4.2 (condition D), very similar to those obtained for condition A, show that B. derxii strain ICB-10 isolated from an acid soil can survive at pH 4.2 without suffering interference in either parameter measured.
The influence of aluminium on the growth of nitrogen-fixing bacteria depends on the species and on the environment: nitrogen incorporation by a strain of A. chroococcum was altered by 230 µM Al2(SO4)3 at pH 7.1, a strain of Beijerinckia indica was affected only at pH 5.0, and another strain of B. mobilis did not suffer any alteration (8). This lack of susceptibility was attributed to acid tolerance. Nevertheless, the B. derxii tested here (Fig. 2) had its growth pattern partially affected by this metal despite being tolerant to low pH, indicating that Al3+ susceptibility must be independent of pH susceptibility. Renner and Howard (20) noted that Al3+ is able to inhibit nucleotide-dependent switch proteins, such as nitrogenase. However, under the tested conditions of this study, nitrogenase activity was restricted to the exponential growth phase and was not affected by the presence of aluminium (Table 1). One possible explanation for the fact that no influence of Al3+ on specific nitrogenase activity was observed may be the following: genetic and metabolic changes that occur during the stationary phase (13) probably made the cells more susceptible to the presence of aluminium. Indeed, the fact that an early death phase was observed corroborates with the above hypothesis.
Although growth of B. derxii strain ICB-10 may be limited by low phosphate concentration (3), higher concentrations were not propitious to its growth, reducing µmax at both 50 mM and 100 mM potassium phosphate and with a low n at 100 mM when compared to condition A (Fig. 3 and Table 1). Becking (8) reported that growth was proportional to the phosphate concentration up to certain levels that were lower for B. indica and B. mobilis than for A. chroococcum; a proportional decrease in growth was observed at higher phosphate concentrations. Working with Klebsiella pneumoniae, Bergersen (9) proposed that the phosphate requirements of N2-fixing systems are not higher than those of systems utilizing combined N.
The ability of B. derxii strain ICB-10 to adapt to the low pH, excess of aluminium and scarcity of phosphate that predominate in lateritic soils is unquestionable: growth at pH 4.2 was similar to condition A, low alterations were observed at elevated aluminium concentrations and low phosphate concentration improved bacterial growth. In all cited situations, specific nitrogenase activity was not affected, suggesting the presence of mechanisms that simultaneously deal with these three conditions.
The comparison of still cultures (condition H) showed that shaking (condition A) improved cell growth (Figs. 1 and 4). Despite the ability of B. derxii strain ICB-10 to produce a slime layer both under still and shaken conditions, under the conditions of this study, nitrogenase was not totally protected against the action of O2 (1). Aerobic nitrogen fixers fix nitrogen more efficiently at sub-atmospheric oxygen tension. In still cultures the gradual increase of specific nitrogenase activity to elevated values, during a phase where no growth was occurring, indicates that the lower redox potencial of the medium (21) promoted conditions for continuous nitrogenase activity.
Studies conducted with purified nitrogenase showed that sodium thiosulphate functions as an iron-protein reducing agent (10). The present investigation showed that this substance is able to affect nitrogenase activity even inside the living cell; also all the growth parameters studied were altered (Table 1 and Fig. 5). The nitrogenase activity increased 70-fold, suggesting that Na2S2O3 may act on the cell in a manner similar to its action on the purified enzyme, increasing the reducing power available for enzyme catalysis, even though other metabolic activities had been affected (growth inhibition).
B. derxii strain ICB-10 may be differently affected by distinct environments. However, no relationship between any affected growth parameter and specific nitrogenase activity was found, suggesting that different mechanisms may be triggered depending on the environmental conditions.
Under some adverse conditions to growth (pH 2.8, low O2 availability, and presence of sodium thiosulphate), stimulation of specific nitrogenase activity occurred. This effect is probably related to the enzymatic regulation resulting in an increased synthesis or stimulation of nitrogenase activity. When a bacterial population is affected by a certain substance, an effective metabolic regulation is possibly triggered in order to preserve the particularly relevant molecules under such conditions. In Anabaena doliolum, copper caused different effects like a decrease of about 50% in specific growth rate, dry weight and lipid concentration, but only a 12% decrease in protein concentration and stimulation of the ability to synthesize pigments (19). However, Nostoc linckia, under cadmium stress, presented delayed nitrogenase activity proportional to the agent concentration (12).
The present report shows that certain environmental factors which depress growth may stimulate nitrogenase during the exponential, stationary and death growth phases. The stimulus may be very high during the stationary or death phases, conditions in which bacteria are commonly found in the environment. Preservation of nitrogenase activity under these environmental conditions emphasizes the importance of this enzyme for nitrogen-fixing organisms.
ACKNOWLEDGEMENTS
The authors thank FUNDAP and CNPq for undergraduate fellowships.
Submited: October 30, 2001. Returned to authors for corrections: April 08, 2002. Approved: september 24, 2002.
- 1. Barbosa, H.R.; Struckel, Y.F. Slime production by Beijerinckia derxii effects of cultural conditions. Soil Biol. Biochem., 17: 731-732, 1985.
- 2. Barbosa, H.R.; Struckel, Y.F.; Almeida-Filho, R.C. Nitrogenase activity of a negative nitrate reductase Beijerinckia derxii grown in a carbon less medium. Rev. Microbiol, 17: 264-269, 1986.
- 3. Barbosa, H.R.; Garcia-Canedo, A.M.G. A concentração de fosfato no crescimento de Beijerinckia derxii cultivada com N2 ou NH4 como fonte de nitrogênio. R. Bras. Ci. Solo, 13: 399-402, 1989.
- 4. Barbosa, H.R.; Alterthum, F. The role of extracellular polysaccharide in cell viability and nitrogenase activity of Beijerinckia derxii. Can. J. Microbiol, 38: 986-988, 1992.
- 5. Barbosa, H.R.; Rodrigues, M.F.A.; Campos, C.C.; Chaves, M.E., Nunes, I.; Juliano, Y.; Novo, N.F. Counting of viable cluster-forming and non cluster-forming bacteria: a comparison between the drop and the spread methods. J. Microbiol Methods, 22: 39-50, 1995.
- 6. Bearson, S.; Bearson, B.; Foster, J.W. Acid stress responses in enterobacteria. FEMS Microbiol. Lett, 147: 173-180, 1997.
- 7. Becking, J.H. Studies on nitrogen-fixing bacteria of the genus Beijerinckia I. Geographical and ecological distribution in soils. Plant Soil., XIV: 49-81, 1961.
- 8. Becking, J.H. Studies on nitrogen-fixing bacteria of the genus Beijerinckia II. Mineral nutrition and resistance to high levels of certain elements in relation to soil type. Plant Soil, XIV: 297-322, 1961b.
- 9. Bergersen, J.F. The effects of phosphate concentration on N2 and NH4-grown Klebsiella pneumoniae. J. Gen. Microbiol, 84: 412-414, 1974.
- 10. Burris, R.H., Nitrogenases. J. Biol. Chem., 266: 9339-9342, 1991.
- 11. Dalton, H.; Postgate J.R. Effect of oxygen on growth of Azotobacter chroococcum in batch and continuous cultures. J. Gen. Microbiol., 54: 463-473, 1969.
- 12. Husaini, Y.; Rai, L.C. Studies on nitrogen and phosphorus metabolism and the photosynthetic electron transport system of Nostoc linckia under cadmium stress. J. Plant Physiol., 138: 429-435, 1991.
- 13. Kolter, R.; Siegele, D.A.; Tormo, A. The stationary phase of the bacterial life cycle. Ann. Rev. Microbiol., 47: 855-874, 1993.
- 14. Langenbach, T.; Nascimento, A.; Sarpa, M. Influence of heavy metals on nitrogen fixation and growth of Azospirillum strains. Rev . Lat-amer. Microbiol, 30: 139-142, 1988.
- 15. Malavolta, E.; Sarruge, J.R.; Bittencourt, V.C. Toxidez de alumínio e de manganês. In: VI Simpósio sobre o Cerrado São Paulo, Ed. Universidade de São Paulo, p.275-301, 1976.
- 16. Pham, D.N.; Burgess, B.K. Nitrogenase reactivity: effects of pH on substrate reduction and CO inhibition. Biochemistry, 32: 13725-13731, 1993.
- 17. Pirt, S.J. Principles of microbial cell cultivation. Wiley, New York., 1975, 274p.
- 18. Postgate, J.R., Nitrogen Fixation. Cambridge University Press, Cambridge, 1998, 112p.
- 19. Rai, L.C.; Mallick, N.; Singh, J.B.; Kumar , H.D. Physiological and biochemical characteristics of a copper tolerant and a wild type strain of Anabaena doliolum under copper stress. J. Plant Physiol, 138: 68-74, 1991.
- 20. Renner, K.A.; Howard, J.B. Aluminum fluoride inhibition of nitrogenase: stabilization of a nucleotide center dot Fe-protein center dot MoFe-protein complex. Biochemistry, 35: 5353-5358, 1996.
- 21. Trolldenier, G. Influence of some environmental factors on nitrogen fixation in the rhizosphere of rice. Plant Soil, 47: 203-217, 1977.
- 22. Trivedi, N.C.; Tsuchiya, H.M. Microbial mutualism in leaching of Cu-Ni sulfide concentrate. Intern. J. Min. Processing, 2: 1-14, 1975.
- 23. Turner, G.L.; Gibson A.H. Measurement of nitrogen fixation by indirect means. In: Methods for Evaluating Biological Nitrogen Fixation (F.J. Bergersen, Ed.), Wiley, Chichester, 1980, p.111-138.
- 24. Tsuchiya, H.M.; Trivedi, N.C.; Schuler, M.L. Microbial mutualism in ore leaching. Biotech. Bioeng, XVI 991-995, 1974.
Publication Dates
-
Publication in this collection
23 June 2003 -
Date of issue
Sept 2002
History
-
Accepted
24 Sept 2002 -
Received
30 Oct 2001 -
Reviewed
08 Apr 2002