Abstracts
BACKGROUND: Reperfusion of the skeletal muscle worsens existing lesions during ischemia, since the production of reactive oxygen species, associated with intense participation of neutrophils, increases the inflammatory reaction that induces tissue changes. OBJECTIVE: To evaluate the morphological and immunohistochemical changes of the skeletal (soleus) muscle of rats submitted to ischemia and reperfusion with pentoxifylline. METHODS: Sixty rats were submitted to ischemia of the pelvic limb for 6 hours induced by clamping the left common iliac artery. After ischemia, group A animals (n = 30) were observed for 4 hours and group B animals (n = 30) for 24 hours. Six animals constituted the sham group. Pentoxifylline was applied only in the reperfusion period A2 (n = 10) and B2 (n = 10), and in ischemia and reperfusion periods in A3 (n = 10) and B3 (n = 10). The soleus muscle was evaluated by histological (fiber disruption, leukocyte infiltrate, necrosis) and immunohistochemical (apoptosis through caspase-3 expression) analysis. The non-parametric tests Kruskal-Wallis and Mann-Whitney (p < 0.05) were applied. RESULTS: The changes were more intense in group B1, with fiber disruption mean scores of 2.16±0.14; neutrophilic infiltrate of 2.05±0.10; and caspase-3 expression in the perivascular area of 4.30±0.79; and less intense in group A3, with means of 0.76±0.16; 0.92±0.10; 0.67±0,15, respectively (p < 0.05). Caspase-3 was more expressive in group B1 in the perivascular area, with mean of 4.30±0.79 when compared with group B1 in the perinuclear area, with mean of 0.91±0.32 (p < 0.05) CONCLUSIONS: The lesions were more intense when observation time was longer after reperfusion, and pentoxifylline attenuated these lesions, above all when used in the beginning of ischemia and reperfusion phases.
Ischemia; reperfusion; skeletal muscle; rats; pentoxifylline; caspases; apoptosis
CONTEXTO: A reperfusão de músculo esquelético piora as lesões já presentes no período de isquemia, pois a produção de espécies reativas de oxigênio, associadas à intensa participação de neutrófilos, amplia a reação inflamatória que induz alterações teciduais. OBJETIVO: Avaliar as alterações morfológicas e imuno-histoquímicas de músculo esquelético (sóleo) de ratos submetidos a isquemia e reperfusão com pentoxifilina. MÉTODOS: Sessenta ratos foram submetidos a isquemia do membro pélvico, por 6 horas, pelo clampeamento da artéria ilíaca comum esquerda. Após isquemia, os animais do grupo A (n = 30) foram observados por 4 horas, e os do grupo B (n = 30), por 24 horas. Seis animais constituíram o grupo simulado. Administrou-se pentoxifilina apenas no período de reperfusão em A2 (n = 10) e B2 (n = 10) e nos períodos de isquemia e reperfusão em A3 (n = 10) e B3 (n = 10). O músculo sóleo foi avaliado por análise histológica (dissociação de fibras, infiltrado leucocitário, necrose) e imuno-histoquímica (apoptose pela expressão da caspase-3). Foram aplicados os testes não-paramétricos de Kruskal-Wallis e Mann-Whitney (p < 0,05). RESULTADOS: As alterações foram mais intensas no grupo B1, com médias de escore da dissociação de fibras musculares de 2,16 ± 0,14, infiltrado neutrofílico de 2,05 ± 0,10 e expressão da caspase-3 na área perivascular de 4,30 ± 0,79; e menos intensas no grupo A3, com respectivas médias de 0,76 ± 0,16, 0,92 ± 0,10 e 0,67 ± 0,15 (p < 0,05). A caspase-3 mostrou-se mais expressiva no grupo B1 na área perivascular, com média de 4,30 ± 0,79, em comparação com o grupo B1 na área perinuclear, com média de 0,91 ± 0,32 (p < 0,05). CONCLUSÕES: As lesões são mais intensas quando o tempo de observação é maior após a reperfusão, e a pentoxifilina atenua essas lesões, sobretudo quando usada no início das fases de isquemia e de reperfusão.
Isquemia; reperfusão; músculo esquelético; ratos; pentoxifilina; caspases; apoptose
ORIGINAL ARTICLE
Ischemia and reperfusion of the soleus muscle of rats with pentoxifylline
José Lacerda BrasileiroI; Djalma José FagundesII; Luciana Odashiro Nakao MiijiIII; Celina Tizuko Fujiama OshimaIV; Roberto TeruyaV; Guido MarksV; Celso Massaschi InouyeV; Maldonat Azambuja SantosVI
IMember, SBACV. Preceptor, Medical Residence in Vascular Surgery, Hospital Universitário, Universidade Federal de Mato Grosso do Sul (UFMS), Campo Grande, MS, Brazil
IIAssociate professor, Operative Technique and Experimental Surgery, Universidade Federal de São Paulo Escola Paulista de Medicina (UNIFESPEPM), São Paulo, SP, Brazil
IIIMSc. Professor, Pathology, UFMS, Campo Grande, MS, Brazil
IVProfessor, Laboratory of Molecular Pathology, Department of Pathology, UNIFESPEPM, São Paulo, SP, Brazil
VProfessors, Department of Surgical Clinic, UFMS, Campo Grande, MS, Brazil
VICoordinator, Commission for Medical Residence (COREME), Hospital Universitário, UFMS, Campo Grande, MS, Brazil. Supervisor, Medical Residence in Vascular Surgery, Hospital Universitário, UFMS, Campo Grande, MS, Brazil
Correspondence Correspondence: José Lacerda Brasileiro Rua Gonçalo Alves, 59, Vivendas do Bosque CEP 79021182 Campo Grande, MS Tel.:(67) 9281.8940, (67) 3326.2842 Email: jlbrasileiro@brturbo.com.br
ABSTRACT
BACKGROUND: Reperfusion of the skeletal muscle worsens existing lesions during ischemia, since the production of reactive oxygen species, associated with intense participation of neutrophils, increases the inflammatory reaction that induces tissue changes.
OBJECTIVE: To evaluate the morphological and immunohistochemical changes of the skeletal (soleus) muscle of rats submitted to ischemia and reperfusion with pentoxifylline.
METHODS: Sixty rats were submitted to ischemia of the pelvic limb for 6 hours induced by clamping the left common iliac artery. After ischemia, group A animals (n = 30) were observed for 4 hours and group B animals (n = 30) for 24 hours. Six animals constituted the sham group. Pentoxifylline was applied only in the reperfusion period A2 (n = 10) and B2 (n = 10), and in ischemia and reperfusion periods in A3 (n = 10) and B3 (n = 10). The soleus muscle was evaluated by histological (fiber disruption, leukocyte infiltrate, necrosis) and immunohistochemical (apoptosis through caspase3 expression) analysis. The nonparametric tests KruskalWallis and MannWhitney (p < 0.05) were applied.
RESULTS: The changes were more intense in group B1, with fiber disruption mean scores of 2.16 ± 0.14; neutrophilic infiltrate of 2.05 ± 0.10; and caspase3 expression in the perivascular area of 4.30 ± 0.79; and less intense in group A3, with means of 0.76 ± 0.16; 0.92 ± 0.10; 0.67 ± 0,15, respectively (p < 0.05). Caspase3 was more expressive in group B1 in the perivascular area, with mean of 4.30 ± 0.79 when compared with group B1 in the perinuclear area, with mean of 0.91±0.32 (p < 0.05).
CONCLUSIONS: The lesions were more intense when observation time was longer after reperfusion, and pentoxifylline attenuated these lesions, above all when used in the beginning of ischemia and reperfusion phases.
Keywords: Ischemia, reperfusion, skeletal muscle, rats, pentoxifylline, caspases, apoptosis.
Introduction
Reperfusion of the skeletal muscle, although necessary to revert ischemic state, worsens existing lesions during the ischemic period, since, due to oxygen coming and production of reactive oxygen species associated with intense participation of neutrophils, it increases the inflammatory reaction, with consequent morphological changes, such as interstitial and cellular edema, leukocyte infiltrate, microcirculation thrombosis, apoptosis or tissue necrosis.16
The soleus muscle has been used to study oxidative changes because type I fibers are prevalent in its constitution (91%), being considered a model sensitive to oxidative stress.4,7 However, to cause ischemia of the soleus muscle, one should consider that vascularization of the pelvic limb in rats has an exuberant presence of collateral circulation in the external iliac artery and common femoral artery; thus, these aspects should be considered when choosing the model to be used to cause ischemia.7,8 In the model of ischemia induced by isolated arterial clamping, direct compression of the venous bed is avoided, as well as the direct muscle trauma caused by the model that uses a tourniquet, allowing preservation of vasomotor and venous responses during reperfusion.2,8
Pentoxifylline has proved to be promising. It is a standard hemorheologic drug to be used in chronic vascular diseases that has been tested to modulate morphological and biochemical changes caused by ischemia and reperfusion in many organs and tissues, such as heart,9 ovary,10 muscle,11,12 liver,13 lung,14 spinal cord15 and intestine.16 It acts as phosphodiesterase inhibitor, which induces increase in cyclic adenosine monophosphate, improving microcirculation flow; reduces migration of neutrophils; reduces release of cytokines; increases production of prostacyclines; and reduces release of reactive oxygen species. These properties have been responsible for its attenuating action on ischemic and reperfusion lesion.6,9,16,17
This study aims at developing a model of ischemia and reperfusion in rats to assess morphological and immunohistochemical changes of the soleus muscle with pentoxifylline.
Method
This research project was approved by the Research Ethics Committee of Universidade Federal de Mato Grosso do Sul (UFMS) and Escola Paulista de Medicina da Universidade Federal de São Paulo (UNIFESPEPM), according to the research protocols 38/2003 and 0334/04. All animals were handled in accordance with the ethical principles of animal experimentation prescribed by the World Society for the Protection of Animals and Federal Act 663.
Sixtysix Wistar EPM1, male and adult rats (Rattus norvegicus albinus) were used, mean weight of 335.6 g, taken from the vivarium at UFSM, where operative procedures were carried out.
The animals were randomly distributed into simulated group (SG), group A and group B, according to the types of experimental procedure:
SG (n = 6): animals submitted to anesthesia, laparotomy, with no ischemia of the upper limb and collection of soleus muscle after 4 hours (n = 3) and 24 hours (n = 3) of observation;
Group A (n = 30): animals submitted to anesthesia, laparotomy, 6hour ischemia of the posterior limb and collection of soleus muscle after 4 hours of reperfusion;
Group B (n = 30): animals submitted to anesthesia, laparotomy, 6hour ischemia of the posterior limb and collection of soleus muscle after 24 hours of reperfusion.
The animals in groups A and B were redistributed into subgroups 1 (A1 and B1), 2 (A2 and B2) and 3 (A3 and B3) according to application of pentoxifylline:
A1 and B1 (n = 10): animals that were not given the drug, whether during ischemia period, whether during reperfusion period;
A2 and B2 (n = 10): animals that were not given the drug only immediately before reperfusion (3 minutes);
A3 and B3 (n = 10): animals that were not given the drug both immediately before ischemia (3 minutes) and immediately before reperfusion (3 minutes).
The animals were anesthetized using intraperitoneal injection of 2:1 solution of ketamine (50 mg.mL1) and xylazine chloride (20 mg.mL1), respectively, at 0.1 mL.100g1. Nalbuphine (Nubain®) was subcutaneously administered at 2 mg.kg1 for analgesia while the animals were under observation, during ischemia or reperfusion periods. Pentoxifylline (Trental®) at 40 mg.kg1 was intraperitoneally administered in a 2 mL volume. Median laparotomy was performed to insert a vascular microclamp for a 6hour period in the left common iliac artery (Figure 1).
Vascular microclamp was removed after 6 hours of ischemia, for observation according to groups of 4 and 24 hours of reperfusion with water and diet ad libitum.
After desired reperfusion period, euthanasia was performed by total exsanguination of the animal, causing death due to hypovolemia.
The muscle was dissected and excised (Figure 2) for morphological and immunohistochemical study. The tissue was submerged in buffered formol (for each liter of 10% formol, 4 g anhydrous monobasic sodium phosphate and 6.5 g anhydrous dibasic sodium phosphate were added) for morphological (hematoxylineosin) and immunohistochemical (caspase3 expression) assessment. Processing and analysis of optical microscopy were performed by a pathologist from the Department of Pathology at UNIFESPEPM, without previous knowledge of experimental groups. Immunochemical processing and quantification were performed at the Laboratory of Molecular Pathology of the Department of Pathology.
In the analysis by optical microscopy, magnification of 100 and 400 x, hematoxylineosinstained sections were observed in relation to the following morphological aspects of muscle cells: dissociation of muscle fibers (degree of tissue edema), presence of neutrophilic infiltrate (inflammatory response) and destruction of the sarcolemma (cellular necrosis).
With the aim of allowing comparison between groups, a system of parameter (scores) graduation was established (Table 1): for each parameter, the arithmetic average of each value was calculated for the 20 fields assessed in each section, corresponding to one animal of each group.
Immunohistochemical processing was performed using primary antibody cleaved anticaspase3, antirat (DAKO® A/S, Denmark 492), DAB staining (3,3diaminobenzidine) Sigma CoUSAD5632 and obtaining brownish caspase3 expression.
Immunohistochemical quantification was performed by capturing 10 images per section of each animal, using an optical microscope Nikon®, connected to a microcomputer Pentium® III, 650 MHz, 256 MB Ram, 40 GB HD and operational system Microsoft Windows® 98 SE.
Quantification of the brownish density was performed using an RGB filter, blue background, color interval between zero and 147, which corresponds to the brown spectrum.1820 Analysis was performed using a computer software of image analysis (ImageLAB® 2.3), based on spectrophotometry principles.19 To quantify each parameter, 10 microscopic fields were captured, with marked spots (standardized size of 3 cm) in the perinuclear and perivascular areas of the muscle fiber, representative of apoptosis of cell nuclei of interest in each image.
For each tissue, corresponding to one animal from each group, the arithmetic average of the values in each area was calculated (perivascular and perinuclear) in the 10 fields in relation to the nonstained area by immunohistochemistry, and expressed in percentages (Figure 3).
Statistical study was performed using KruskalWallis and MannWhitney tests to compare subgroups. P values < 0.05 were considered statistically significant, with 95% confidence interval.
Results
Morphological analysis by optical microscopy
The dissociation of muscle fibers, interstitial neutrophilic infiltrate and necrosis of muscle fibers were the parameters analyzed by optical microscopy. Despite being investigated, the variable necrosis was not found in this experimental model. After morphological analysis, score averages were calculated for each assessed variable in all subgroups.
There were no changes in these parameters in the SG; for that reason, this group could not be compared to experimental subgroups.
Results of average calculations and respective standard deviations in scores for the subgroups are shown in Tables 2 Click to enlarge and 3 Click to enlarge and in Figures 4 and 5 .
Morphological description of dissociation of fibers
Separation of muscle fibers can be interpreted as being caused by interposition of interstitial fluid and, therefore, an expression of edema and muscle tissue swelling.
The muscle of SG animals, whether being 4 or 24 hours under investigation, did not show separation of muscle fibers, which presented its characteristic fusiform disposition and uniform alignment in the whole assessed field.
Group A3 animals and some group B3 animals were those that presented the most favorable scores, with predominance of mild separation degree, characterized by the presence of one spacing per field and the rest of fusiform fibers uniformly aligned.
The animals in groups A1 and A2 and some in group B3 presented the most representative scores of moderate dissociation of fibers, in which two to three spaces were identified in each field, and the other fibers maintained the fusiform characteristic, but already presented some misalignment with tortuosity. The animals in groups B1 and B2 presented severe degree of dissociation of fibers, characterized by several spaces between fibers, which were tortuous and misaligned.
Morphological description of leukocyte infiltrate
The presence of inflammatory cells in the interstitium was characterized as an indicator of intensity in inflammatory cell response of the muscle tissue to ischemia and reperfusion.
The muscle of SG animals, whether being 4 or 24 hours under investigation, did not show leukocyte infiltrate between muscle fibers, which presented its characteristic fusiform disposition and uniform alignment in the whole assessed field.
The animals in group A3 and some in group B3 were those that presented the most favorable scores, with predominance of mild degree of leukocyte infiltrate, characterized by presence of one to 10 cells per field, usually close to the vessels in intercellular spaces.
The animals in groups A1 and A2 and some in group B3 were those that presented the most representative scores of moderate leukocyte infiltrate, in which between 10 and 20 inflammatory cells infiltrated in the intercellular space and in farther spaces of the vascular space.
The animals in groups B1 and B2 presented severe degree of neutrophilic infiltrate, characterized by many cells, more than 20 per field, between muscle fibers.
Immunohistochemistry results
Immunostaining was assessed by caspase3 expression activated in perivascular and perinuclear areas of the muscle fiber. A standard 3 x 3 cm square area was developed to establish the chosen region for caspase3 expression in immunostaining percentage of respective areas, using an imageLab® computer software.
The results of means, in percentage, of caspase3 expression of all groups are shown in Tables 4 Click to enlarge , 5 Click to enlarge and 6 Click to enlarge and in Figures 6 , 7 and 8 .
The use of pentoxifylline assessed in the perinuclear area during reperfusion, whether at an early stage (A2 = 0.39 ± 0.11) or at a late stage (B2 = 0.47 ± 0.21), did not show differences; however, in both cases the expression was significantly lower in the animals that were not given the drug. When its application was assessed in both periods (ischemia and reperfusion), at an early (A3 = 0.27 ± 0.12) or late (B3 = 0.37 ± 0.15) stage, both cases presented results significantly lower than the animals that were not given pentoxifylline, although there were no differences at the moment the drug was applied. Therefore, pentoxifylline was equally favorable in manifestation of apoptosis in perinuclear neutrophils both at an early and late stage, showing immediate and sustained activity.
Comparison of caspase expression between perinuclear and perivascular areas in different administration periods and assessment stages (Figure 8 ) shows that pentoxifylline was significantly favorable in reducing apoptosis in both areas with higher intensity when administered in both periods (ischemia and reperfusion).
Discussion
In case there is ischemia of lower limbs, priority should be reestablishing blood flow. Extension and severity of tissue morphological and functional changes essentially depend on the period of occlusion of arterial blood flow.16
Studies using experimental animals have tried to assess models that may be able to reproduce ischemic conditions found in human beings and, therefore, have a better understanding of the disease physiopathology and therapeutic alternatives.2,21,22
We chose the model of isolated obstruction of the common iliac artery because it meets the objectives of attempting to simulate occurrence of ischemia resulting from acute arterial obstructions, which often occur in medical practice.8 Projectpilot tests showed that edema and cyanosis presented by the pelvic limb of the animals after arterial clamping were reliable parameters to confirm ischemia of this limb.
The literature is consensual as to the soleus muscle being the most appropriate for studying lesions resulting from ischemia and reperfusion,4,7,23,24 since the type of fiber and location of muscle groups are related to the degree of lesion caused by ischemia and reperfusion. The soleus muscle, because it has 91% of type I fibers, is more sensitive to ischemic processes. Its higher sensitivity to oxidative stress, resulting from the production of reactive oxygen species, is explained by its metabolic route to obtain energy, preferentially oxidation of glycerides and, at a lower degree, the glycogenic oxidative route.4,7
The skeletal muscle is deemed resistant to ischemic lesions, despite the specific sensitivity of the soleus muscle.4,7,23,24 The literature shows that the skeletal muscle supports ischemia well for up to 4 hours, despite presenting some local and systemic repercussions.
Morphological lesions start manifesting around 15 minutes after ischemia (reperfusion) and progressively increase as the inflammatory response increases. They are more evident with 4 hours and reach maximum peak after 24 hours.21,25,26 Therefore, we tried to study changes considered early (4 hours) and late (24 hours). Periods previous to 4 hours bring irrelevant results, and periods longer then 24 hours show that there is a tendency of lesion reduction.25
One of the first signs occurring after arterial deobstruction is represented by interstitial edema. Extravasation of intravascular fluids into the interstitium is caused by biophysical (mechanical) changes, by increased intracapillary pressure, and later by biochemical changes, by increased capillary permeability, which result in accumulation of fluids between muscle fibers, leading to dissociation of these fibers. The larger the edema, the larger the dissociation of fibers, with presence of a temporal relationship: the longer the ischemic period, the larger the edema.6,27,28 Infiltration of interstitial fluid separates muscle fibers, and the examination of representative fields using the same microscopic magnification could characterize the degree of this infiltration.
The ischemic process triggers an expected inflammatory response, characterized by diapedesis of leukocytes that are interposed, from vascular spaces and between muscle fibers. At first, leukocyte concentration takes place around the vessels and, as the process advances, is spread into the interstitium of muscle fibers. Neutrophils are more frequently found within 24 hours. They represent an organism's response to humoral processes triggered by the inflammatory process, aiming to isolate the aggressive factor and start the process of lesion repair.6,27,24,29,30 Besides edema and leukocyte infiltration and as a consequence of absence of oxygen or oxidizable substrate, muscle cells may gradually progress to necrosis.24
Caspase3 expression can be used as a marker of apoptosis. Caspases, which belong to the protease family, are cysteine proteases activated by factors released by the mitochondria in the process of cell death and can be identified by immunohistochemical process.3,18,27
Marking muscle fibers (perinuclear) aimed at identifying apoptosis through caspase3 expression specifically on the skeletal muscle tissue. Marking neutrophils in the perivascular areas was an attempt to express the magnitude of the inflammatory reaction. The higher the leukocyte suffering, expressed by apoptosis in neutrophils, the most severe the lesion originated from ischemia and reperfusion.3,18,27
Forms of abolishing or minimizing the effects of reactive oxygen species or free radicals have been investigated.5,21,23,24,31,32 For that, substances inhibiting formation of free radicals have been used, known as antioxidants (vitamin E, ascorbic acid, deferoxamine, streptokinase, allopurinol, magnesium salts, aminosteroids, iron chelators and nonsteroid antiinflammatory drugs, scavengers)31 and processes of ischemic preconditioning, in which partial and progressive suppressions of oxygenation antecede a sustained but prolonged suppression.5,31 Each of these propositions have shown advantages and disadvantages in dependence of ischemia and reperfusion models, in doses of substances used, in periods in which they are administered, in parameters that are used to assess results, among other variables.6
Pentoxifylline has antioxidating action, directly inhibiting the superoxide anion and indirectly blocking the action of xanthine oxidase. In addition, it seems to reduce inflammatory response by reducing migration of neutrophils and other mechanisms, reducing release of cytokines, tumor necrosis factor, plateletactivating factor and release of endothelin, which is a potent vasoconstrictive substance.9,10,17 Considering these theoretical supports, use of this drug has been proposed to test its activity in ischemia and reperfusion of the skeletal muscle.
Analysis and interpretation of histological results, concerning assessed parameters, showed some significant variations that deserved attention.
SG showed that anesthetic and operative trauma had no significant influence on muscle tissue and can be used as a comparison point with the other two study groups. The findings obtained were considered as normality pattern.
Absence of morphological changes typical of tissue necrosis was significant. Muscle fibers in animals submitted to 6hour ischemia did not show visible necrotic reactions 4 and 24 hours after reestablishment of tissue perfusion. This fact may demonstrate the resistance of muscle tissue against ischemic aggression, but it should also be associated with the capacity or peculiarity of collateral arterial circulation of posterior limbs in rats and with the nonimpairment of venous reflux.
Some authors mentioned in the literature, who used the same arterial clamping time in rats, failed in identifying areas of muscle fiber necrosis, although they had observed the occurrence of other morphological changes and increased creatinine phosphokinase, a parameter indicating necrosis.2.21
Dissociation of fibers, which expresses presence of interstitial edema in animals observed after 4 hours of reperfusion, showed that application of pentoxifylline during reperfusion was not different from that in animals of the control group. However, when the drug was administered in two periods (ischemia and reperfusion), there was significant reduction in edema expression. The profile was repeated in the 24hour observation, when the control group was similar to the group receiving the drug in reperfusion and significantly lower in the group given the drug in both periods. Therefore, it can be concluded that pentoxifylline acted on the process, improving edema expression. Since the initial component of intravascular fluid loss is mechanical (biophysical), due to increased intracapillary pressure, and later biochemical, involving cell vitality and enzymatic activities that lead to increased vascular patency and force relationship of tissue osmotic tension, it can be inferred that the hemorheologic capacity of pentoxifylline should interfere favorably, avoiding or making intravascular fluid loss difficult.
Relationship of tissue edema is timedependent. Within 4 hours of reperfusion, the edema is less important than within 24 hours. At early and late stages, pentoxifylline proved to be efficacious in reducing edema. One could suppose that, because it reduced the edema at the early stage, this represents a consequent effect when observing the late stage, but, since drug metabolization is slow, it can be inferred that there is still effective drug activity at a late stage as well.
Studies report the relationship of progressive increase in morphological changes in a 24hour period after ischemia in the skeletal muscle.25,26 After that period, there is a tendency to normalization of tissues proportional to observation time after ischemia.25
The same theoretical relationship about edema can be established to assess migration of neutrophils. It is known that pentoxifylline is able to inhibit migration of leukocytes.9,14,15,17 Since leukocyte migration is an active process, dependent on local release of active substances, such as cytokines, tumor necrosis factor, platelet and endothelin activating factor, and these humoral factors are modulated by the drug, it is reasonable to infer that its activity was also favorable in this aspect on ischemia and muscle reperfusion lesion.
The results of this research are in agreement with others in the biomedical literature. A study on clamping of the abdominal aorta in rats for 90 minutes and reperfusion for 60 minutes showed that pentoxifylline mitigated edema and leukocyte infiltrate in the pelvic limb muscles of the animals when used in ischemic and reperfusion periods.12 In another model of skeletal muscle ischemia for 5 hours and postischemia observation for 20 hours, it was possible to conclude that, the higher the pentoxifylline dose, the better the protective effects by edema reduction, leukocyte infiltrate and areas of muscle fibers, as well as by reduction in platelet activating factor.11 In another experimental model of ischemia in pelvic limbs of rats by clamping of the abdominal aorta, it was observed that local and pulmonary repercussion in the group that was given pentoxifylline had reduction in levels of alpha tumor necrosis factor (TNFa) and leukocyte infiltrate in the muscle and lung, when compared to the control group and to the group receiving dexametasone.33
The suggestion of analyzing caspase3 expression in perivascular and perinuclear areas of muscle fiber was based on literature studies that found higher evidence of apoptosis only in neutrophilic infiltrate and vessels of muscles submitted to oxidative stress, showing that muscle fiber is more resistant to apoptosis and more sensitive to necrosis.27,34,35
Assessment of apoptosis through immunohistochemical caspase3 expression showed that it has little importance on the normal muscle tissue, represented by animals in the SG. Expression in neutrophils in perinuclear area proved to be discrete, when compared to perivascular area, and both were much lower than the values of animals submitted to ischemia and reperfusion. Therefore, expression of apoptosis in neutrophils in SG conditions, in which there was only influence of anesthetic and operative trauma, was practically inexistent.
However, expression in the animals submitted to ischemia and reperfusion in perivascular area, at early or late stage, is significantly higher, as well as in perinuclear area, both in early and in late stages. Expression was timedependent in perivascular area, where, at an early stage, the index was lower than in a late stage. In perinuclear area, the indexes were equivalent, both at early and late stages. These findings allow us to infer that perinuclear neutrophils and muscle fiber nuclei are less susceptible of starting an apoptosis process than perivascular neutrophils, revealing that the latter can be more directly involved in processes of tissue lesion or more exposed to aggressive factors of ischemic and reperfusion lesion. The main aggressive activating factor of caspase cascade is attributed to release of reactive oxygen species during reperfusion.18,27,34
Pentoxifylline proved to be efficacious in reducing caspase3 expression, which is a monitor of cell apoptosis, when there was sum of doses applied in both periods.
Pentoxifylline, in this experimental model, proved to be efficacious in attenuating the inflammatory reaction by reducing edema and leukocyte infiltrate and in reducing caspase3 expression, which places it according to reports in the literature about its mechanisms of action on ischemia and reperfusion.
The findings of this research allows us to conclude that pentoxifylline is a drug that should continue being part of the therapeutic options that have been investigated to abolish or attenuate ischemic and reperfusion lesions and that the model proposed in this study is feasible and adds a new assessment parameter of ischemic and reperfusion lesions through caspase3 expression in leukocyte infiltrate of the muscle tissue.
Conclusions
The ischemia and reperfusion model in the soleus muscle of rats produced morphological changes, timedependent of the reperfusion period, but with no evidence of necrosis or muscle apoptosis.
Pentoxifylline proved to be favorable in attenuating morphological changes in the muscle tissue, by reducing edema and neutrophilic infiltrate between muscle fibers, when applied at the start of ischemia and reperfusion.
It also proved to be favorable in attenuating caspase3 expression, in leukocyte infiltrate in perivascular and perinuclear areas, being more significant when applied at the start of ischemia and reperfusion.
References
Manuscript received November 30, 2006, accepted February 27, 2007.
This research was carried out at the Graduate Program in Surgery and Experimentation, UNIFESPEPM, São Paulo, SP, Brazil.
- 1. Silva JCCB, Burihan E. Diagnóstico clínico da isquemia crítica dos membros. Rev Bras Clin Ter. 1999;25:71-9.
- 2. Francisco Neto A, Silva JCCB, Fagundes DJ, et al. Estudo das alterações oxidativas, da capacidade antioxidante total e do óxido nítrico, em ratos submetidos à isquemia e reperfusão de membros posteriores. Acta Cir Bras. 2005;20:134-9.
- 3. Lockshin RA, Zakeri Z. Caspase-independent cell deaths. Curr Opin Cell Biol. 2002; 14:727-33.
- 4. Poli Figueiredo LF, Cruz Jr. RJ, Morsch RD. Desvascularização e reperfusão no trauma vascular. In: Murilo XX. Trauma vascular. 1Ş ed. Rio de Janeiro: Revinter; 2005. p. 628-33.
- 5. Bitu-Moreno J, Gregório EA, Maffei FHA. Effect of alpha-tocopherol on the ischemia/reperfusion lesions induced in the hindlimb of rats. Acta Cir Bras. 2001;16:68-74.
- 6. Silveira M, Yoshida WB. Isquemia e reperfusão em músculo esquelético: mecanismos de lesão e perspectivas de tratamento. J Vasc Bras. 2004;3:367-78.
- 7. Badhwar A, Dungey AA, Harris KA, et al. Limitations of ischemic tolerance in oxidative skeletal muscle: perfusion vs tissue protection. J Surg Res. 2003;109:62-7.
- 8. Quiñones-Baldrich WJ, Chervu A, Hernandez JJ, Colburn M, Moore WS. Skeletal muscle function after ischemia: "no reflow" versus reperfusion injury. J Surg Res.1991;51:5-12.
- 9. Zhang M, Xu YJ, Saini HK, Turan B, Liu PP, Dhalla NS. Pentoxifylline attenuates cardiac dysfunction and reduces TNF-alpha level in ischemic-reperfused heart. Am J Physiol Heart Circ Physiol. 2005;289:H832-9.
- 10. Uguralp S, Bay Karabulut A, Mizrak B. Effects of pentoxifylline and vitamin E on the bilateral ovary after experimental ovarian ischemia. Eur J Pediatr Surg. 2005;15:107-13.
- 11. Adams JG Jr., Dhar A, Shukla SD, Silver D. Effect of pentoxifylline on tissue injury and platelet-activating factor production during ischemia-reperfusion injury. J Vasc Surg. 1995;21:742-8; discussion 748-9.
- 12. Kishi M, Tanaka H, Seiyama A, et al. Pentoxifylline attenuates reperfusion injury in skeletal muscle after partial ischemia. Am J Physiol. 1998;274(5 Pt 2):H1435-42.
- 13. Yildirim S, Tok H, Koksal H, Erdem L, Baykan A. Allopurinol plus pentoxifylline in hepatic ischaemia/reperfusion injury. Asian J Surg. 2002;25:149-53.
- 14. Clark SC, Rao JN, Flecknell PA, Dark JH. Pentoxifylline is as effective as leukocyte depletion for modulating pulmonary reperfusion injury. J Thorac Cardiovasc Surg. 2003;126:2052-7.
- 15. Dominguez-Jimenez C, Sancho D, Nieto M, et al. Effect of pentoxifylline on polarization and migration of leukocytes. J Leukoc Biol. 2002;71:588-96.
- 16. Sener G, Akgun U, Satiroglu H, Topaloglu U, Keyer-Uysal M. The effect of pentoxifylline on intestinal ischemia/reperfusion injury. Fundam Clin Pharmacol. 2001;15:19-22.
- 17. Schratzberger P, Dunzendorfer S, Reinisch N, et al. Mediator-dependent effects of pentoxifylline on endothelium for transmigration of neutrophils. Immunopharmacology. 1999;41:65-75.
- 18. Gown AM, Willingham MC. Improved detection of apoptotic cells in archival paraffin sections: Immunohistochemistry using antibodies to cleaved caspase 3. J Histochem Cytochem. 2002;50:449-54.
- 19. Borra RC, Pizarro PJ. Imagelab98. Disponível em: http//www.brasil.terravista.pt/PraiaBrava/1563/imgelab.html Acessado: 04/04/2005.
- 20. Kohlberger PD, Breitenecker F, Kaider A, et al. Modified true-color computer-assisted image analysis versus subjective scoring of estrogen receptor expression in breast cancer: a comparison. Anticancer Res. 1999;19:2189-93.
- 21. Souza-Moraes MR, David-Filho R, Baptista-Silva JC, et al. Effect of antibodies to intercellular adhesion lmolecule type 1 on the protection of distant organs during reperfusion syndrome in rats. Braz J Med Biol Res. 2003;36:605-12.
- 22. Fagundes DJ, Taha MO. Modelo animal de doença: critérios de escolha e espécies de animais de uso corrente. Acta Cir Bras. 2004;19:59-65.
- 23. Francischetti I, Maffei FHA, Bitu-Moreno J, et al. Ação do ácido trissódio-cálcio-dietileno-triaminopentaacético (CaNa3DTPA) nas lesões de isquemia-reperfusão em membro posterior de rato. Acta Cir Bras. 2002;17:1-17.
- 24. Torres JMS, Guimarães SB, Vasconcelos PRL, Martins MCR, Chaves CR, Vasconcelos PRC. Efeitos metabólicos da l-alanil-glutamina em ratos submetidos à isquemia da pata traseira esquerda seguida de reperfusão. Acta Cir Bras. 2003;18:39-44.
- 25. Wiersema AM, Oyen WJ, Dirksen R, Verhofstad AA, Corstens FH, van der Vliet JA. Early assessment of skeletal muscle damage after ischaemia-reperfusion injury using Tc-99m-glucarate. Cardiovasc Surg. 2000;8:186-91.
- 26. Lazarus B, Messina A, Barker JE, et al. The role of mast cells in ischaemia-reperfusion injury in murine skeletal muscle. J Pathol. 2000;191:443-8.
- 27. Cowled PA, Leonardos L, Millard SH, Fitridge RA. Apoptotic cell death makes a minor contribution to reperfusion injury in skeletal muscle in the rat. Eur J Vasc Endovasc Surg. 2001;21:28-34.
- 28. Souza AC, Piccinato CE, Cherri J, Moriya T. Mecanismos celulares e moleculares da lesão de isquemia e reperfusão de músculo esquelético. Cir Vasc Angiol. 2001;17:80-6.
- 29. Piccinato CE, De Domenico Jr. A, Jordäo Jr. AA, Vannucchi H. Skeletal muscle ischemia and reperfusion in rats increase lipid peroxidation in rats. Acta Cir Bras. 2004;19:578-81.
- 30. Alves WF, Guimaräes SB, Vasconcelos PRC, Vasconcelos PRL. Repercussões da L-alanil-glutamina sobre as concentrações de lactato e lactato desidrogenase (LDH) em pacientes com isquemia crítica dos membros inferiores submetidos à revascularização distal. Acta Cir Bras. 2003;18:209-15.
- 31. Bitu-Moreno J, Francischetti I, Hafner L. Lesöes de isquemia-reperfusão em músculos esqueléticos: fisiopatologia e novas tendências de tratamento, com ênfase em reperfusão controlada. J Vasc Bras. 2002;1:113-20.
- 32. David Filho R, Moraes MRS, Silva JCCB, et al. Blockage of intracellular adhesion molecule-1 (ICAM-1) in the prevention of reperfusion lesion in the skeletal musculature of EPM-1 Wistar rats. Acta Cir Bras. 2004;19:590-6.
- 33. Berkan O, Gol MK, Icagasioglu S, et al. Sialic acid is a marker of lung injury following lower extremities ischemia/reperfusion. Eur J Vasc Endovasc Surg. 2004;27:553-8.
- 34. Knight KR, Messina A, Hurley JV, Zhang B, Morrison WA, Stewart AG. Muscle cells become necrotic rather than apoptotic during reperfusion of ischaemic skeletal muscle. Int J Exp Pathol.1999;80:169-75.
- 35. Leeuwenburgh C, Gurley CM, Strotman BA, Dupont-Versteegden EE. Age-related differences in apoptosis with disuse atrophy in soleus muscle. Am J Physiol Regul Integr Comp Physiol. 2005;288:R1288-96.
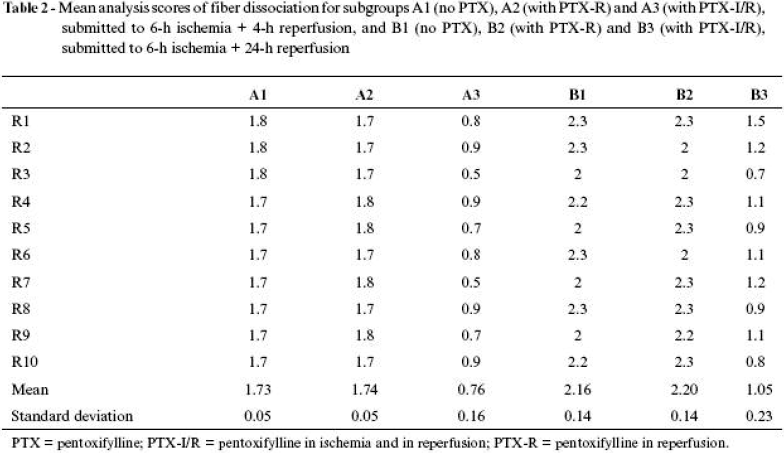
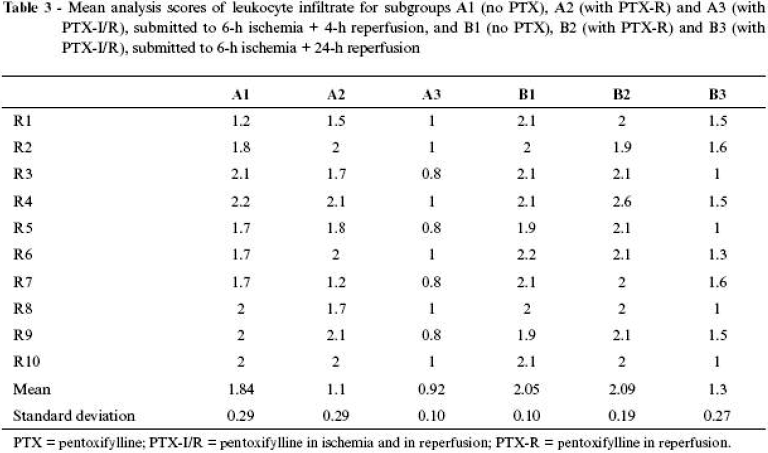
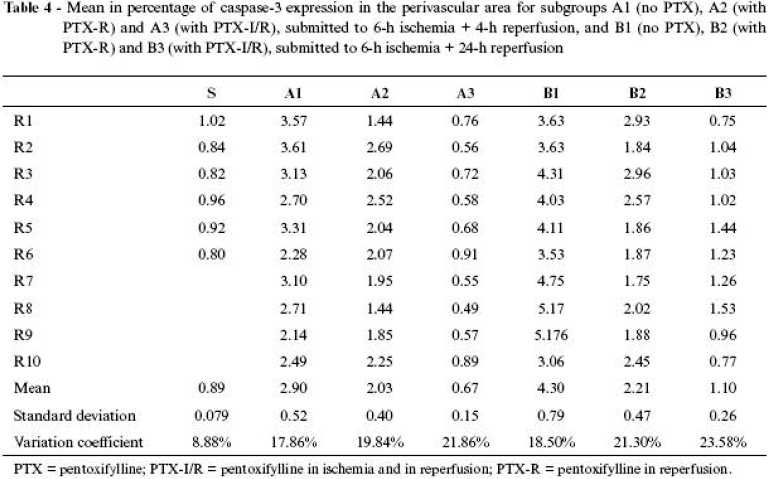
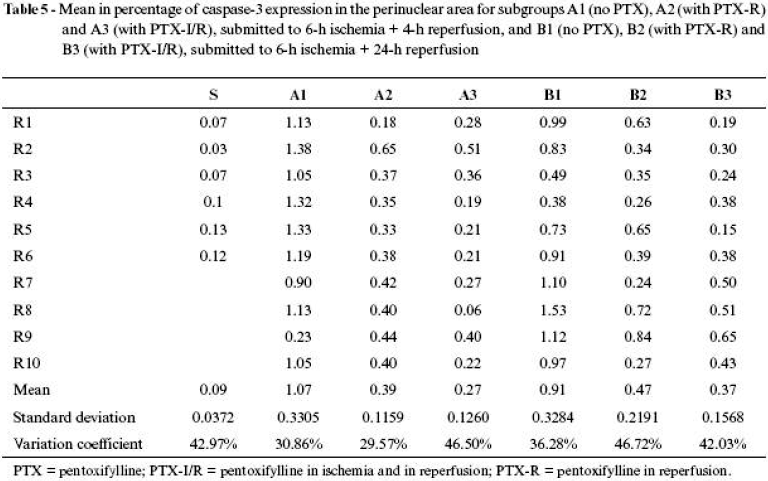
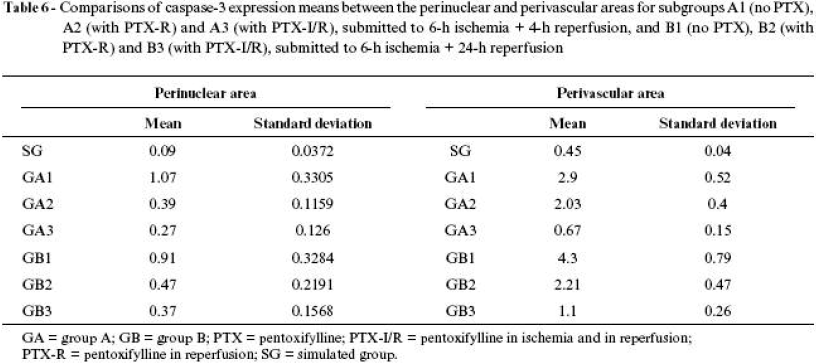
Correspondence:
Publication Dates
-
Publication in this collection
19 July 2007 -
Date of issue
Mar 2007
History
-
Accepted
27 Feb 2007 -
Received
30 Nov 2006