Abstract
A specific, sensitive and robust LC–MS/MS method was developed and validated for the quantification of deoxyelephantopin in rat plasma using simvastatin as an internal standard as per regulatory guidelines of Bioanalytical Method Validation. Plasma sample was prepared through liquid-liquid extraction. Chromatographic separation was performed on an Agela-C18 analytical column (1.8 µm, 2.1 mm × 50 mm) with an isocratic mobile phase consisting of 0.05% formic acid (dissolved in acetonitrile) and water (55:45, v/v) at a flow rate of 0.5 ml/min. The column oven was maintained at 40 ºC and the injection volume was 4 µl. Elution of deoxyelephantopin and the internal standard occurred at 5.1 and 6.3 min, respectively. The total chromatographic run time was 7.5 min. A linear response function was constructed in the concentration range of 13.2–2640 ng/ml. The intra- and inter-day precision and accuracy were in the range of 1.4–14.8% and –11.7 to 14.1%, respectively. The validated LC–MS/MS was successfully applied to the pharmacokinetic study of deoxyelephantopin after intravenous injection of 1, 2 and 4 mg/kg and oral administration of 7.5, 15 and 30 mg/kg deoxyelephantopin in rats. After oral and intravenous administration, the C max and AUC values of deoxyelephantopin increased dose-dependently.
Keywords
Deoxyelephantopin; Pharmacokinetics; LC–MS/MS; Rat plasma
Introduction
Elephantopus scaber L., Asteraceae, is widely used in folk medicines for the treatment of arthritis, hepatitis, diarrhea, diabetes, leukemia and eczema in many countries (Behera and Misra, 2005Behera, S.K., Misra, M.K., 2005. Indigenous phytotherapy for genitor-urinary diseases used by the Kandha tribe of Orissa. J. Ethnopharmacol. 102, 319-325.; Rajakumar and Shivanna, 2010Rajakumar, N., Shivanna, M.B., 2010. Traditional herbal medicinal knowledge in Sagar taluk of Shimoga district, Karnataka, India. Indian J. Nat. Prod. Resour. 1, 102-108.). Recent pharmacological studies have demonstrated that E. scaber possesses various medicinal properties including antidiabetic, anticancer, hepatoprotective, antibacterial, anti-inflammatory, and anti-asthmatic abilities (Ho et al., 2012Ho, W.Y., Yeap, S.K., Ho, C.L., Abdul Rahim, R., Alitheen, N.B., 2012. Hepatoprotective activity of Elephantopus scaber on alcohol-induced liver damage in mice. Evid. Based Complement. Alternat. Med., http://dx.doi.org/10.1155/2012/417953.
http://dx.doi.org/10.1155/2012/417953...
; Kabeer et al., 2013Kabeer, F.A., Sreedevi, G.B., Nair, M.S., Rajalekshmi, D.S., Gopalakrishnan, L.P., Kunjuraman, S., Prathapan, R., 2013. Antineoplastic effects of deoxyelephantopin, a sesquiterpene lactone from Elephantopus scaber, on lung adenocarcinoma (A549) cells. J. Integr. Med. 11, 269-277.; Chan et al., 2015Chan, C.K., Supriady, H., Goh, B.H., Kadir, H.A., 2015. Elephantopus scaber induces apoptosis through ROS-dependent mitochondrial signaling pathway in HCT116 human colorectal carcinoma cells. J. Ethnopharmacol. 168, 291-304.). These effects are supposed to be attributed to sesquiterpene lactones, the major constituent extracted from E. scaber (Hiradeve and Rangari, 2014Hiradeve, S.M., Rangari, V.D., 2014. Elephantopus scaber Linn.: a review on its ethnomedical, phytochemical and pharmacological profile. J. Appl. Biomed. 12, 49-61.).
Sesquiterpene lactones are natural products with unusual structure that are abundant in many plants and possess a broad spectrum of biological activities, including antifungal, immunomodulatory, anti-proliferative, anti-inflammatory, and antibacterial activities (Hou and Huang, 2016Hou, L., Huang, H., 2016. Immune suppressive properties of artemisinin family drugs. Pharmacol. Ther. 166, 123-127.; Schepetkin et al., 2018Schepetkin, I.A., Kirpotin, L.N., Mitchell, P.T., Kishkentaev, S., Shaimerdenov, Z.R., Atazhanov, G.A., Adekenov, S.M., Quinn, M.T., 2018. The natural sesquiterpene lactones arglabin, grosheimin, agracin, parthenolide, and estafiatin inhibit T cell receptor (TCR) activation. Phytochemistry 146, 36-46.). Deoxyelephantopin (1) is a sesquiterpene lactone component of E. scaber. Previous studies reported that deoxyelephantopin possessed anti-inflammatory, antiprotozoal, antineoplastic and hepatoprotective activities (Singh et al., 2005Singh, S.D.J., Krishna, V., Mankani, K.L., Manjunatha, B.K., Vidya, S.M., Manohara, Y.N., 2005. Wound healing activity of the leafextracts and deoxyelephantopin isolated from Elephantopus scaber Linn.. Indian J. Pharmacol. 37, 238-242.; Kabeer et al., 2013Kabeer, F.A., Sreedevi, G.B., Nair, M.S., Rajalekshmi, D.S., Gopalakrishnan, L.P., Kunjuraman, S., Prathapan, R., 2013. Antineoplastic effects of deoxyelephantopin, a sesquiterpene lactone from Elephantopus scaber, on lung adenocarcinoma (A549) cells. J. Integr. Med. 11, 269-277.; Huang et al., 2013Huang, C.C., Lin, K.J., Cheng, Y.W., Hsu, C.A., Yang, S.S., Shyur, L.F., 2013. Hepatoprotective effect and mechanistic insights of deoxyelephantopin, a phyto-sesquiterpene lactone, against fulminant hepatitis. J. Nutr. Biochem. 24, 516-530.; Zahari et al., 2014Zahari, Z., Jani, N.A., Amanah, A., Latif, M.N., Majid, M.I., Adenan, M.I., 2014. Bioassay-guided isolation of a sesquiterpene lactone of deoxyelephantopin from Elephantopus scaber Linn active on Trypanosome brucei rhodesience. Phytomedicine 21, 282-285.; Andy et al., 2018Andy, S.N., Chan, C.K., Kadir, H.A., 2018. Deoxyelephantopin from Elephantopus scaber modulates neuroinflammatory response through MAPKs and PI3K/Akt- dependent NF-kB signaling pathways in LPS-stimulated BV-2 microglial cells. J. Funct. Foods 38, 221-231.). Recent studies have demonstrated that deoxyelephantopin can induce apoptosis in various cancer cells such as human nasopharyngeal, cervical carcinoma, osteosarcoma, colorectal carcinoma, and lung cancer cells through multiple mechanisms (Su et al., 2011Su, M., Chung, H.Y., Li, Y., 2011. Deoxyelephantopin from Elephantopus scaber L. induces cell-cycle arrest and apoptosis in the human nasopharyngeal cancer CNE cells. Biochem. Biophys. Res. Commun. 411, 342-347.; Lee and Shyur, 2012Lee, W.L., Shyur, L.F., 2012. Deoxyelephantopin impedes mammary adenocarcinoma cell motility by inhibiting calpain-mediated adhesion dynamics and inducing reactive oxygen species and aggresome formation. Free Radic. Biol. Med. 52, 1423-1436.; Farha et al., 2014Farha, A.K., Dhanya, S.R., Mangalam, S.N., Geetha, B.S., Latha, P.G., Remani, P., 2014. Deoxyelephantopin impairs growth of cervical carcinoma SiHa cells and induces apoptosis by targeting multiple molecular signaling pathways. Cell Biol. Toxicol. 30, 331-343., 2015Farha, A.K., Dhanya, S.R., Mangalam, S.N., Remani, P., 2015. Anti-metastatic effect of deoxyelephantopin from Elephantopus scaber in A549 lung cancer cells in vitro. Nat. Prod. Res. 29, 2341-2345.; Zou et al., 2017Zou, J., Zhang, Y., Sun, J., Wang, X., Tu, H., Geng, S., Liu, R., Chen, Y., Bi, Z., 2017. Deoxyelephantopin induces reactive oxygen species-mediated apoptosis and autophagy in human osteosarcoma cells. Cell Physiol. Biochem. 42, 1812-1821.). Given these remarkable functions, more attention has been paid to the pharmacological research on deoxyelephantopin and its clinical therapeutic application.
Different analytical methods have been described for the qualitative and quantitative determination of deoxyelephantopin in herbal materials or related preparations, including thin-layer chromatography (Wu et al., 2014Wu, J., Sun, L., Wang, J., 2014. Qualitative and quantitative analysis of deoxyelephantopin in Elephantopus scaber L.. Anhui Med. Pharm. J. 18, 1862-1865.), high- performance liquid chromatography with a diode array detector (Wu et al., 2013Wu, C., Xiao, J., Wu, X., Wang, G., Zhou, G., Li, Y., 2013. Fingerprint study and content determination of three active components of Elephantopus scaber L.. Lishizhen Med. Mater. Med. Res. 24, 1643-1645., 2014Wu, J., Sun, L., Wang, J., 2014. Qualitative and quantitative analysis of deoxyelephantopin in Elephantopus scaber L.. Anhui Med. Pharm. J. 18, 1862-1865.; Zhao et al., 2015Zhao, A.Q., Zhao, J.H., Zhang, S.Q., Pan, Y.Y., Huo, X.L., 2016. Determination of parthenolide in rat plasma by UPLC–MS/MS and its application to a pharmacokinetic study. J. Pharm. Biomed. Anal. 119, 99-103.), and gas chromatography (Anees et al., 2009Anees, A., Alkarkhi, A.F.M., Hena, S., Khim, L.H., 2009. Extraction, separation and identification of chemical ingredients of Elephantopus scaber L. using factorial design of experiment. Int. J. Chem. 1, 36-49.). However, the sensitivity of these methods is not sufficient for quantitative analysis of deoxyelephantopin in biological matrix. In the present study, a simple and sensitive LC–MS/MS method was developed for the quantification of deoxyelephantopin in rat plasma. The method was fully validated with respect to the FDA guidelines for the validation of bioanalytical methods. Finally, it was successfully applied to the pharmacokinetic study of deoxyelephantopin after oral and intravenous administrations to rats.
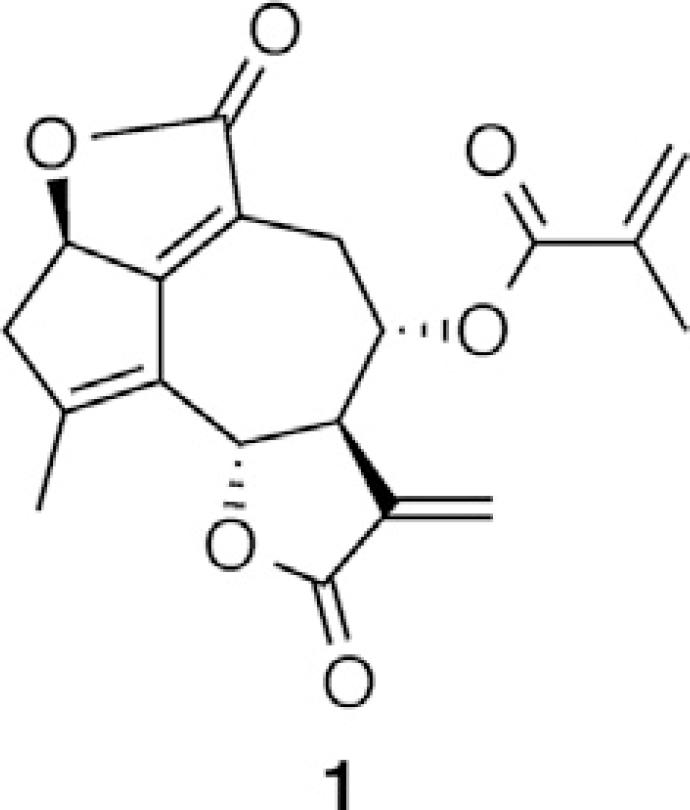
Materials and methods
Chemicals and reagents
Deoxyelephantopin (1) (99.0% purity) was purchased from Chengdu Ruifensi Co., Ltd (Chengdu, China), and simvastatin (100% purity) used as an internal standard (IS) was purchased from the National Institutes for Food and Drug Control (Beijing, China). HPLC-grade acetonitrile and methanol were purchased from Merck (Darmstadt, Germany), and HPLC-grade formic acid was acquired from Tedia (Fairfield, OH, USA). Ethyl acetate (analytical reagent grade) was purchased from Sinopharm Chemical Reagent Co., Ltd (Shanghai, China). Water was purified obtained from Milli-Q purification system (Millipore, Bedford, MA, USA) and filtered through a 0.22 µm membrane filter (MF-Mixed Cellulose Ester Membrane Filter, Millipore, Bedford, MA, USA) before use.
Liquid chromatographic and mass spectrometric conditions
Samples were performed using a TSQ Triple-Quadrupole mass spectrometer with an electrospray ionization (ESI), coupled to an Ultimate 3000 high-performance liquid chromatography system controlled by the Xcalibur software (Thermo Scientific, San Jose, USA). Chromatographic separation of deoxyelephantopin was conducted by using an Agela-C18 analytical column (1.8 µm, 2.1 mm × 50 mm, Agela Technologies, USA) with an isocratic mobile phase consisting of 0.05% formic acid (dissolved in acetonitrile) and water (55:45, v/v) at a flow rate of 0.5 ml/min. The column oven and autosampler were maintained at 40 ºC and 15 ºC, respectively. The total analytical run time was 7.5 min.
The ESI source was operated in the positive ion mode. Tuning parameters were optimized for both deoxyelephantopin (1) and internal standard (IS). Finally, the ion source conditions were set as follows: capillary voltage, 4.0 kV; ion transfer capillary temperature, 350 ºC; 45 arbitrary units; auxiliary gas, 10 arbitrary units; scan time was set at 0.2 s. Nitrogen was used as sheath gas and auxiliary gas. Argon was used as the collision gas and the pressure of collision cell was 1.5 mTorr. Selected reaction monitoring (SRM) was used for data acquisition. The optimized SRM fragmentation transitions were m/z 367→279 with a collision energy of 40 eV for deoxyelephantopin, and m/z 441→325 with a collision energy of 28 eV for IS.
Preparation of calibration standards and Quality Control (QC) samples
A stock solution of deoxyelephantopin was prepared at a concentration of 660 µg/ml and serially diluted with methanol to yield working solutions. A stock solution of IS was prepared at a concentration of 1.35 mg/ml and diluted with methanol to yield a working solution of 135 ng/ml. Seven non-zero calibration standards (plasma matrix processed with analyte and IS) and three QC samples were prepared by spiking an aliquot of deoxyelephantopin working solution into blank rat plasma at a ratio of 1:19 (v/v) at 13.2, 52.8, 132, 264, 528, 1320, and 2640 ng/ml for calibration samples, and 39.6, 211.2, and 2112 ng/ml for QC samples. All working solutions and calibration standards were stored at –20 ºC and brought to room temperature before use.
Sample preparation
To an aliquot (50 µl) of rat plasma, 20 µl of the IS working solution and 1 ml of ethyl acetate were added and vortex-mixed for 3 min. After centrifugation at 12,300 × g for 5 min, the clear supernatant (700 µl) was separated and evaporated to dryness at 45 ºC under a stream of nitrogen. The residue was dissolved in 100 µl of the mobile phase, and then vortex-mixed. A 4 µl aliquot was injected into the LC–MS/MS system for analysis.
Method validation
The method was validated for selectivity, sensitivity, linearity, precision and accuracy, matrix effect, recovery, and stability in accordance with the US FDA guidelines for the validation of bioanalytical methods (US Food and Drug Administration, 2001US Food and Drug Administration, 2001. Guidance for Industry: Bioanalytical Method Validation, http://www.fda.gov/downloads/Drugs/Guidance/ucm070107.pdf (accessed 10.01.17).
http://www.fda.gov/downloads/Drugs/Guida...
). The selectivity was checked by comparing chromatograms of blank plasma from six different rats, blank plasma spiked with deoxyelephantopin and IS, and plasma samples obtained after administration of deoxyelephantopin. Linearity was estimated from the calibration curve correlation coefficient R
2 with an acceptance criterion defined as R
2 > 0.99. Sensitivity was evaluated by determining the LLOQ (lower limit of quantification), using the signal-to-noise ratio (S/N) of 10. Recovery was assessed by comparing processed QC samples with reference deoxyelephantopin solutions in blank plasma extract at the same levels. Matrix effect was determined by comparing the reference solutions in blank rat plasma extracts with corresponding free-matrix solutions at the three QC levels. Precision was expressed in terms of the percentage of the coefficient of variation (%CV), and accuracy was expressed in terms of the percentage of relative error (%RE), calculated from the difference between the measured concentration and the theoretical value. Bench-top (at room temperature for 6 h), autosampler (in-injector for 24 h), three freeze-thaw cycle, and long term stability (at -20 ºC for 50 days) were performed at three QC levels using six replicates.
Pharmacokinetic study
The method was applied to a pharmacokinetic study of deoxyelephantopin (1) in rats. The rats were maintained on a 12 h light–dark cycle with free access to food and water for a week. Before the experiment, all rats were fasted for 12 h and had free access to water, and then orally given deoxyelephantopin at 7.5, 15 and 30 mg/kg or intravenously administered at 1, 2 or 4 mg/kg, respectively (n = 6 SD rats for each group). Approximately 250 µl of blood samples were collected from the oculi chorioideae vein into heparinized tubes at 0, 0.17, 0.5, 1, 1.5, 2, 3, 5, 7, 9, 12 and 24 h after oral dosing and at 0, 0.08, 0.17, 0.33, 0.67, 1, 1.5, 2, 3, 5, 7, and 9 h after intravenous injection. Blood samples were centrifuged at 1120 × g for 10 min, and the plasma was obtained. The plasma samples were labeled and kept frozen at –20 ºC until analysis.
Results and discussion
LC–MS/MS condition optimization
Deoxyelephantopin (1) and IS were investigated for the abundant precursor ions [M+Na]+ at m/z 367 and 441, respectively. The quantification of analyte was chosen using SRM mode for high sensitivity and selectivity of acquisition data. It is found that the fragmentation ion at m/z 279 and m/z 325 were the prominent product ions for deoxyelephantopin and IS, respectively (Fig. 1). Therefore, the transitions of 367→279 and 441→325 for were selected for quantification of deoxyelephantopin and IS.
To achieve symmetric peak shapes and short run times of deoxyelephantopin and IS, the Agela-C18 analytical column (1.8 µm, 2.1 mm × 50 mm) and mobile phase consisted of 0.05% formic acid (dissolved in acetonitrile)-water (55:45, v/v) were selected for analysis. Typical chromatograms of blank plasma and plasma spiked with deoxyelephantopin and IS were presented in Fig. 2. No significant endogenous substance was observed at the retention times of deoxyelephantopin and IS in blank rat plasma.
Representative SRM chromatograms of a blank rat plasma sample (A), a blank rat plasma sample spiked with deoxyelephantopin at LLOQ and IS (B), a rat plasma sample obtained at 0.5 h after oral administration of deoxyelephantopin (30 mg/kg) (C), and a rat plasma sample obtained at 2 h after intravenous administration of deoxyelephantopin (4 mg/kg) (D). R t = 5.1 min, deoxyelephantopin; R t = 6.3 min, IS.
Method validation
Calibration curve, accuracy, and precision
The equations of calibration curves were constructed by using a weighted (1/x 2) least-squares linear regression analysis. All calibration curves of the analyte showed excellent linearity over the studied concentration range (13.2–2640 ng/ml). The correlation coefficients (r 2) of all equations were ≥0.995, and the deviation of each point on calibration curve were less than ±8.6%. The regression equations for calibration curves were as follows: y = 5.295 × 10–6 x + 9.985 × 10–5 (r 2 = 0.9982). The LLOQ value for deoxyelephantopin was 13.2 ng/ml that showed a good sensitivity. In comparison to the previous method (Zhao et al., 2015Zhao, W., Liu, N., Lei, J., 2015. Simultaneous determination of schaftoside, isoschaftoside, deoxyelephantopin and 4,5-dicaffeoylquinic acid in shennongcha granules by HPLC. Chin. Pharm. 18, 741-744.), the sensitivity of the current LC–MS/MS method was significantly improved and the LLOQ was decreased from 4.16 µg/ml to 13.2 ng/ml. The method was appropriated to the quantitative analysis and pharmacokinetic study of deoxyelephantopin in plasma samples. The details of intra- and inter-day accuracy and precision are presented in Table 1. The results demonstrated that the LC–MS/MS method was sensitive, accurate and reproducible.
Intra- and inter-day precision and accuracy determination of deoxyelephantopin in rat plasma.
Stability, recovery and matrix effect
The percentage mean recovery for deoxyelephantopin at all QC levels were found to be 90.9 ± 1.5, 85.4 ± 5.2 and 89.1 ± 2.1%, respectively (n = 6). The mean percentage recovery (mean ± SD; n = 6) of IS was found to be 82.0 ± 3.6%. Matrix effect study indicates that no co-eluting substance could influence the ionization of deoxyelephantopin and IS.
Stability data under all storage conditions tested are summarized in Table 2, suggesting that the compound is stable for the routine LC–MS/MS analysis.
Pharmacokinetic study
The applicability of the assay was demonstrated in the pharmacokinetic study after intravenous injection of 1, 2 and 4 mg/kg and oral administration of 7.5, 15 and 30 mg/kg deoxyelephantopin in rats. The mean plasma concentration-time profiles after oral administration and intravenous administration are shown in Fig. 3. Meanwhile, the major pharmacokinetic parameters are summarized in Table 3.
Mean (±SD) plasma concentration–time profiles of deoxyelephantopin after oral (A) administration of deoxyelephantopin (7.5, 15, 30 mg/kg) and intravenous (B) administration of deoxyelephantopin (1, 2, 4 mg/kg) to six rats.
Pharmacokinetic parameters of deoxyelephantopin after intravenous (i.v.) and oral (p.o.) administrations at three doses.
For the oral administration, deoxyelephantopin was rapidly absorbed (Tmax = 1.6–2.0 h), reaching a mean maximum plasma concentration (Cmax), which was calculated to be 63.4 ± 14.8, 109.8 ± 18.5, and 196.9 ± 34.6 ng/ml for 7.5, 15 and 30 mg/kg, respectively. Results indicated that deoxyelephantopin was absorbed rapidly in rats after oral administration, which could be due to its low-polarity characteristic and small molecule size. The elimination half-life (t 1/2) for oral administration of deoxyelephantopin in rats was 4.7 ± 0.7, 4.9 ± 1.5, and 5.7 ± 3.1 h, respectively.
For the intravenous administration, the t 1/2 of deoxyelephantopin in rats was 1.0 ± 0.2, 2.0 ± 0.8, and 2.5 ± 0.4 h for 1, 2 and 4 mg/kg, respectively. The mean t 1/2 values of deoxyelephantopin for oral groups were about 2–5 times greater than those of intravenous groups, indicating that deoxyelephantopin was slowly eliminated in rats following oral administration, but rapidly after intravenous administration.
For the intravenous and oral administrations, the mean C max and AUC values increased dose-dependently. Results indicated that the pharmacokinetics of deoxyelephantopin appeared to increase proportionally from 7.5 to 30 mg/kg, and from 1 to 4 mg/kg.
Compared with the published data describing the pharmacokinetic profiles of other sesquiterpene lactones in rats (Lachi-Silva et al., 2015Lachi-Silva, L., Sy, S.K.B., Voelkner, A., Sousa, J.P.B., Lopes, J.L.C., Silva, D.B., Lopes, N.P., Kimura, E., Derendorf, H., Diniz, A., 2015. Simultaneous characterization of intravenous and oral pharmacokinetics of lychnopholide in rats by transit compartment model. Planta Med. 81, 1121-1127.; Zhao et al., 2016Zhao, A.Q., Zhao, J.H., Zhang, S.Q., Pan, Y.Y., Huo, X.L., 2016. Determination of parthenolide in rat plasma by UPLC–MS/MS and its application to a pharmacokinetic study. J. Pharm. Biomed. Anal. 119, 99-103.), the pharmacokinetic parameters of deoxyelephantopin in the study were generally similar. To the best of our knowledge, this study firstly clarified the pharmacokinetic behavior of deoxyelephantopin in rats. The method presented here is suitable for pharmacokinetic studies and allows to determine the noncompartmental and compartmental pharmacokinetic parameters of deoxyelephantopin after both oral and intravenous administrations.
Conclusions
In the present study, a simple, specific and robust LC–MS/MS method was developed and validated for the quantification of deoxyelephantopin, a novel anti-tumor candidate, in rat plasma for the first time. The method provided good linearity with a low LLOQ (13.2 ng/ml) and was successfully applied to pharmacokinetic studies of deoxyelephantopin after oral and intravenous administration in rats. The reported pharmacokinetic parameters of deoxyelephantopin would provide helpful information for further development.
Ethical disclosures
-
Protection of human and animal subjects. The authors declare that the procedures followed were in accordance with the regulations of the relevant clinical research ethics committee and with those of the Code of Ethics of the World Medical Association (Declaration of Helsinki).
-
Confidentiality of data. The authors declare that they have followed the protocols of their work center on the publication of patient data.
-
Right to privacy and informed consent. The authors declare that no patient data appear in this article.
References
- Anees, A., Alkarkhi, A.F.M., Hena, S., Khim, L.H., 2009. Extraction, separation and identification of chemical ingredients of Elephantopus scaber L. using factorial design of experiment. Int. J. Chem. 1, 36-49.
- Andy, S.N., Chan, C.K., Kadir, H.A., 2018. Deoxyelephantopin from Elephantopus scaber modulates neuroinflammatory response through MAPKs and PI3K/Akt- dependent NF-kB signaling pathways in LPS-stimulated BV-2 microglial cells. J. Funct. Foods 38, 221-231.
- Behera, S.K., Misra, M.K., 2005. Indigenous phytotherapy for genitor-urinary diseases used by the Kandha tribe of Orissa. J. Ethnopharmacol. 102, 319-325.
- Chan, C.K., Supriady, H., Goh, B.H., Kadir, H.A., 2015. Elephantopus scaber induces apoptosis through ROS-dependent mitochondrial signaling pathway in HCT116 human colorectal carcinoma cells. J. Ethnopharmacol. 168, 291-304.
- Farha, A.K., Dhanya, S.R., Mangalam, S.N., Geetha, B.S., Latha, P.G., Remani, P., 2014. Deoxyelephantopin impairs growth of cervical carcinoma SiHa cells and induces apoptosis by targeting multiple molecular signaling pathways. Cell Biol. Toxicol. 30, 331-343.
- Farha, A.K., Dhanya, S.R., Mangalam, S.N., Remani, P., 2015. Anti-metastatic effect of deoxyelephantopin from Elephantopus scaber in A549 lung cancer cells in vitro Nat. Prod. Res. 29, 2341-2345.
- Ho, W.Y., Yeap, S.K., Ho, C.L., Abdul Rahim, R., Alitheen, N.B., 2012. Hepatoprotective activity of Elephantopus scaber on alcohol-induced liver damage in mice. Evid. Based Complement. Alternat. Med., http://dx.doi.org/10.1155/2012/417953
» http://dx.doi.org/10.1155/2012/417953 - Hiradeve, S.M., Rangari, V.D., 2014. Elephantopus scaber Linn.: a review on its ethnomedical, phytochemical and pharmacological profile. J. Appl. Biomed. 12, 49-61.
- Hou, L., Huang, H., 2016. Immune suppressive properties of artemisinin family drugs. Pharmacol. Ther. 166, 123-127.
- Huang, C.C., Lin, K.J., Cheng, Y.W., Hsu, C.A., Yang, S.S., Shyur, L.F., 2013. Hepatoprotective effect and mechanistic insights of deoxyelephantopin, a phyto-sesquiterpene lactone, against fulminant hepatitis. J. Nutr. Biochem. 24, 516-530.
- Kabeer, F.A., Sreedevi, G.B., Nair, M.S., Rajalekshmi, D.S., Gopalakrishnan, L.P., Kunjuraman, S., Prathapan, R., 2013. Antineoplastic effects of deoxyelephantopin, a sesquiterpene lactone from Elephantopus scaber, on lung adenocarcinoma (A549) cells. J. Integr. Med. 11, 269-277.
- Lee, W.L., Shyur, L.F., 2012. Deoxyelephantopin impedes mammary adenocarcinoma cell motility by inhibiting calpain-mediated adhesion dynamics and inducing reactive oxygen species and aggresome formation. Free Radic. Biol. Med. 52, 1423-1436.
- Lachi-Silva, L., Sy, S.K.B., Voelkner, A., Sousa, J.P.B., Lopes, J.L.C., Silva, D.B., Lopes, N.P., Kimura, E., Derendorf, H., Diniz, A., 2015. Simultaneous characterization of intravenous and oral pharmacokinetics of lychnopholide in rats by transit compartment model. Planta Med. 81, 1121-1127.
- Rajakumar, N., Shivanna, M.B., 2010. Traditional herbal medicinal knowledge in Sagar taluk of Shimoga district, Karnataka, India. Indian J. Nat. Prod. Resour. 1, 102-108.
- Schepetkin, I.A., Kirpotin, L.N., Mitchell, P.T., Kishkentaev, S., Shaimerdenov, Z.R., Atazhanov, G.A., Adekenov, S.M., Quinn, M.T., 2018. The natural sesquiterpene lactones arglabin, grosheimin, agracin, parthenolide, and estafiatin inhibit T cell receptor (TCR) activation. Phytochemistry 146, 36-46.
- Singh, S.D.J., Krishna, V., Mankani, K.L., Manjunatha, B.K., Vidya, S.M., Manohara, Y.N., 2005. Wound healing activity of the leafextracts and deoxyelephantopin isolated from Elephantopus scaber Linn.. Indian J. Pharmacol. 37, 238-242.
- Su, M., Chung, H.Y., Li, Y., 2011. Deoxyelephantopin from Elephantopus scaber L. induces cell-cycle arrest and apoptosis in the human nasopharyngeal cancer CNE cells. Biochem. Biophys. Res. Commun. 411, 342-347.
- US Food and Drug Administration, 2001. Guidance for Industry: Bioanalytical Method Validation, http://www.fda.gov/downloads/Drugs/Guidance/ucm070107.pdf (accessed 10.01.17).
» http://www.fda.gov/downloads/Drugs/Guidance/ucm070107.pdf - Wu, J., Sun, L., Wang, J., 2014. Qualitative and quantitative analysis of deoxyelephantopin in Elephantopus scaber L.. Anhui Med. Pharm. J. 18, 1862-1865.
- Wu, C., Xiao, J., Wu, X., Wang, G., Zhou, G., Li, Y., 2013. Fingerprint study and content determination of three active components of Elephantopus scaber L.. Lishizhen Med. Mater. Med. Res. 24, 1643-1645.
- Zahari, Z., Jani, N.A., Amanah, A., Latif, M.N., Majid, M.I., Adenan, M.I., 2014. Bioassay-guided isolation of a sesquiterpene lactone of deoxyelephantopin from Elephantopus scaber Linn active on Trypanosome brucei rhodesience Phytomedicine 21, 282-285.
- Zou, J., Zhang, Y., Sun, J., Wang, X., Tu, H., Geng, S., Liu, R., Chen, Y., Bi, Z., 2017. Deoxyelephantopin induces reactive oxygen species-mediated apoptosis and autophagy in human osteosarcoma cells. Cell Physiol. Biochem. 42, 1812-1821.
- Zhao, W., Liu, N., Lei, J., 2015. Simultaneous determination of schaftoside, isoschaftoside, deoxyelephantopin and 4,5-dicaffeoylquinic acid in shennongcha granules by HPLC. Chin. Pharm. 18, 741-744.
- Zhao, A.Q., Zhao, J.H., Zhang, S.Q., Pan, Y.Y., Huo, X.L., 2016. Determination of parthenolide in rat plasma by UPLC–MS/MS and its application to a pharmacokinetic study. J. Pharm. Biomed. Anal. 119, 99-103.
Publication Dates
-
Publication in this collection
Sep-Oct 2018
History
-
Received
19 Mar 2018 -
Accepted
13 June 2018 -
Published
07 July 2018