Abstract
Five coumarins (5,7,8-trimethoxycoumarin (1), sabandin (2), cubreuva lactone (3), 5,7-dimethoxycoumarin (5) and braylin (6)), seven furoquinoline alkaloids (isopimpinelin (4), pteleine (7), maculine (8), skimianine (10), robustine (11), y-fagarine (12) and dictamine (13) and the furofuran type lignin syringaresinol (9)) have been identified for the first time in the roots of Zanthoxylum tingoassuiba A. St.-Hil., Rutaceae. Pure compounds 1, 6, 9, 12 were tested against Leishmania amazonensis parasites and epimastigotes forms of Trypanosoma cruzi. All the tested products displayed an antiparasitic activity similar to that of the positive controls (benznidazole and amphotericin B). Compound 9 was the most active against both parasites with IC50 values of 11.98 µM and 7.55 µM against L. amazonensis and T. cruzi, respectively.
Keywords
Leismaniasis; Trypansomiasis; Coumarins; Furoquinoline alkaloids; Syringaresinol
Introduction
Leishmaniasis and American trypanosomiasis (Chagas disease) are protozoal parasitic infections with worldwide distribution, which affect mostly sub-developed and developing countries. Both are among the most prevalent neglected tropical diseases (WHO, 2012Tempone, A.G., Treiger Borborema, S.E., de Andrade, H.F., de Amorim Gualda, N.C., Yogi, Á., Salerno Carvalho, C., Bachiega, D., Lupo, F.N., Bonotto, S.V., Fischer, D.C.H., 2005. Antiprotozoal activity of Brazilian plant extracts from isoquinoline alkaloid-producing families. Phytomedicine 12, 382-390.). Chagas disease is caused by the parasite Trypanosoma cruzi and affects approximately 5.7 million people, mainly in Latin America countries (WHO, 2015aWHO, 2012. Research Priorities for Chagas Disease, Human African Trypanosomiasis and Keishmaniasis. WHO Technical Report Series, 116.). Thousands of new cases are reported causing circa 7000 deaths annually, with a significant increase in North America, Europe and Asia (WHO, 2015bWHO, 2015a. Chagas disease in Latin America: an epidemiological update based on 2010 estimates. Wkly. Epidemiol. Record 6, 33-44.). The only drugs used for the treatment of trypanosomiasis are benznidazole and nifurtimox. However, the treatment remains unsatisfactory due to the severe side effects and refractory cases (Paucar et al., 2016Reyes-Chilpa, R., Estrada-Muñiz, E., Vega-Avila, E., Abe, F., Kinjo, J., Hernández-Ortega, S., 2008. Trypanocidal constituents in plants: 7 Mammea-type coumarins. Mem. Inst. Oswaldo Cruz 103, 431-436.). The three different forms of Leishmaniasis (cutaneous, mucocutaneous and visceral) are caused by a parasite of the genus Leishmania. About 12 million people are infected worldwide, with approximately 1 million new cases and 20,000 to 30,000 deaths reported each year (WHO, 2016WHO, 2015b. Investing to Overcome the Global Impact of Neglected Tropical Diseases: Third WHO Report on Neglected Diseases 2015. WHO Document Production Services, Geneva, Switzerland.). The available treatments include pentavalent antimonials, amphotericin B, pentamidine, miltefosine, and paromomycin (Iqbal et al., 2016Iqbal, H., Ishfaq, M., Wahab, A., Abbas, M.N., Ahmad, I., Rehman, A., Zakir, M., 2016. Therapeutic modalities to combat leishmaniasis, a review. Asian Pac. J. Trop. Dis. 6, 1-5.). The emergence of drug resistance, high costs, availability, and toxicity limit the use of these drugs.
New efficient antiparasitic agents are needed, and a great number of works has been developed to discover new bioactive natural compounds (Tagboto and Townson, 2001Tagboto, S., Townson, S., 2001. Antiparasitic properties of medicinal plants and other naturally occurring products. Adv. Parasitol. 50, 199-295.; Kayser et al., 2003Kayser, O., Kiderlen, A.F., Croft, S.L., 2003. Natural products as antiparasitic drugs. Parasitol. Res. 90, S55-S62.; Wink, 2012Wink, M., 2012. Medicinal plants: a source of anti-parasitic secondary metabolites. Molecules 17, 12771-12791.; Cragg and Newman, 2013Cragg, G.M., Newman, D.J., 2013. Natural products: a continuing source of novel drug leads. Biochim. Biophys. Acta Gen. Subj. 1830, 3670-3695.; Annang et al., 2016Annang, F., Genilloud, O., Vicente, F., 2016. Contribution of natural products to drug discovery. In: Tropical Diseases, Comprehensive Analysis of Parasite Biology: From Metabolism to Drug Discovery. Wiley-VCH Verlag GmbH and Co., KGaA, pp. 75–104.; Jain et al., 2016Jain, S., Jacob, M., Walker, L., Tekwani, B., 2016. Screening North American plant extracts in vitro against Trypanosoma brucei for discovery of new antitrypanosomal drug leads. BMC Complement. Altern. Med., http://dx.doi.org/10.1186/s12906-016-1122-0.
http://dx.doi.org/10.1186/s12906-016-112...
). Brazil is known for its high diversity of plant (Mesquita et al., 2005Mesquita, M.L., Desrivot, J., Bories, C., Fournet, A., Paula, J.E., Grellier, P., Espindola, L.S., 2005. Antileishmanial and trypanocidal activity of Brazilian cerrado plants. Mem. Inst. Oswaldo Cruz 100, 783-787.; Tempone et al., 2005Tagboto, S., Townson, S., 2001. Antiparasitic properties of medicinal plants and other naturally occurring products. Adv. Parasitol. 50, 199-295.; Braga et al., 2007Braga, F.G., Bouzada, M.L.M., Fabri, R.L., Matos, M.O., Moreira, F.O., Scio, E., Coimbra, E.S., 2007. Antileishmanial and antifungal activity of plants used in traditional medicine in Brazil. J. Ethnopharmacol. 111, 396-402.; Muzitano et al., 2009Muzitano, M.F., Falcão, C.A.B., Cruz, E.A., Bergonzi, M.C., Bilia, A.R., Vincieri, F.F., Rossi-Bergmann, B., Costa, S.S., 2009. Oral metabolism and efficacy of Kalanchoe pinnata flavonoids in a murine model of cutaneous leishmaniasis. Planta Med. 75, 307-311.; Cechinel Filho et al., 2013Cechinel Filho, V., Meyre-Silva, C., Niero, R., Mariano, L.N.B., Nascimento, F.G., Farias, I.V., Gazoni, V.F., dos Santos Silva, B., Gimenez, A., Gutierrez-Yapu, D., Salamanca, E., Malheiros, A., 2013. Evaluation of antileishmanial activity of selected Brazilian plants and identification of the active principles. Evid. Based Complement Altern. Med., http://dx.doi.org/10.1155/2013/265025.
http://dx.doi.org/10.1155/2013/265025...
; Bou et al., 2014Bou, D.D., Tempone, A.G., Pinto, E.G., Lago, J.H.G., Sartorelli, P., 2014. Antiparasitic activity and effect of casearins isolated from Casearia sylvestris on Leishmania and Trypanosoma cruzi plasma membrane. Phytomedicine 21, 676-681.).
Zanthoxylum genus has been reported to have various bioactivities such as allelopathic, analgesic, anticonvulsant, anti-inflammatory, antimicrobial, antinociceptive, antioxidant, anti helmintic, antiparasitory and antiviral activities, among others. These activities have been related to different chemical constituents: isoquinoline alkaloids, lignans, coumarins, flavonoids and terpenes (Patiño et al., 2012Östan, I., Saglam, H., Limoncu, M.E., Ertabaklar, H., Toz, S.Ö., Özbel, Y., Özbilgin, A., 2007. In vitro and in vivo activities of Haplophyllum myrtifolium against Leishmania tropica. New Microbiol. 30, 439-445.).
Zanthoxylum tingoassuiba A. St.-Hil., Rutaceae, also known as tinguaciba, is one of the 25 species that are endemic to Brazil. The plant is used in folk medicine and had been commercialized in Brazil since 1923 as an active component of a phytotherapeutic formulation prescribed for muscle cramps and spasms (Silva et al., 2008Paucar, R., Moreno-Viguri, Pérez-Silanez, E., 2016. Challenges in chagas disease drug discovery: a review. Curr. Med. Chem. 23, 3154-3170.) and is also marketed as an herbal tea. Our group recently reported its antibacterial activity associated to the presence of benzophenantridine alkaloids (Costa et al., 2017Costa, R.S., Lins, M.O., Le Hyaric, M., Barros, T.F., Velozo, E.S., 2017. In vitro antibacterial effects of Zanthoxylum tingoassuiba bark root extracts and two of its alkaloids against multiresistant Staphylococcus aureus. Rev. Bras. Farmacogn. 27, 195-198.).
Considering the diversity of metabolites produced by plants of the genus Zanthoyxlum and the need for the discovery of new antitrypanosomal and antileishmanial agents, this work aimed to evaluate the antiparasitic activity of compounds isolated from Z. tingoassuiba against L. amazonensis and T. cruzi.
Materials and methods
Plant materials
Zanthoyxlum tingoassuiba A. St.-Hill., Rutaceae, was collected in August 2009 in Jaíba – Feira de Santana, Bahia, Brazil (12º 12′ 52.560″ S; 38º 52′ 46.205″ W). The voucher specimens were identified and deposited at the ALCB – Herbário Alexandre Leal Costa, Instituto de Biologia – UFBA (voucher 88005).
Chemicals
Analytical grade solvents were purchased from Sigma–Aldrich Co. (St. Louis, MO, USA) and used without further purification. HPLC solvents were obtained from Merck (Darmstadt, Germany). Deuterated used for NMR analysis were obtained from CIL (Cambridge Isotope Laboratories Inc., USA).
Instruments
HPLC analysis were performed on a Shimadzu (Kyoto, Japan) Prominence 20A system consisting in a CBM-20A controller, LC-6 AD parallel-flow pumps, DGU-20A degasser, SPD-M20A photodiode array detector (DAD) and a FRC-20A fraction collector. Data were processed by LC Solution Version software (Shimadzu).
The separations of the compound were done on Kromasil C18 (250 × 4.6 mm; 5 µm and 250 × 21 mm; 5 µm) and Phenomenex Luna C18 (250 × 4.6 mm; 5 µm) columns.
HPLC-MS measurements were carried out on a Shimadzu Prominence 20A system consisting in a CBM-20A controller, LC-20 AD parallel-flow pumps, DGU-20A degasser, CTO-20A column oven, SIL-20A autosampler, and DAD-M20A detector. The HPLC system was coupled to a micrOTOF-II mass spectrometer (Bruker Daltonik GmbH, Bremen, Germany)
1H and 13C NMR nuclear magnetic resonance (NMR) spectra were acquired on: Varian Gemini 500 MHz (1H: 500 MHz and 13C: 125 MHz), Bruker DRX400 – Ultra Shield (1H: 400 MHz and 13C: 100 MHz) and Bruker DPX300 (1H: 300 MHz and 13C: 75 MHz) spectrometers, in pyridine-d 5, chloroform-d, acetone-d6 or methanol-d 4 and trimethylsilane (TMS) as an internal standard.
Preparation of the extracts
Root bark (RB) (76.42 g) and of root heartwood (RH) (1.19 g) obtained from Z. tingoassuiba were separated, grinded and extracted four times with methanol (500 ml) by maceration for 7 days and filtered. The filtrates were concentrated under reduced pressure to obtain the root bark methanol extract (RB, 12.72 g) and the root heartwood methanol extract (RH, 54.30 g). The RB extract (11.47 g) was partitioned into 1 l of a mixture CHCl3:MeOH:HCl 5% (5:5:3). The organic phase was separated and evaporated under reduced pressure (RBE1, 3.251 g) and the aqueous phase was basified with ammonium hydroxide until pH 10 was reached. The basic mixture was extracted with chloroform (3 × 300 ml). The organic phase was concentrated under reduced pressure (RBE2, 0.525 g).
The RH methanol extract (30 g) was dissolved in acetic acid 3% (650 ml) and extracted three times with chloroform (3 × 250 ml). The organic phase HR1 was concentrated under reduced pressure (935 mg).
HPLC analysis
RBE1, RBE2 and HR1 were analyzed by HPLC using the following conditions: C18 column (4.6 × 250 mm, 100 Å, 5 µm particle size, Kromasil®), guard column (4.6 × 20 mm, 5 µm particle size), isocratic flow of MeOH:H2O (1:1) at a rate of 0.6 ml min-1, injection volume of 20 µl. The HPLC fractions were monitored with the UV detector at 254 nm.
Preparative HPLC
The RBE1, RBE2 and HR1 fractions were solubilized in methanol (saturated solutions 313.1, 220.1 and 638.0 mg/ml for, respectively) and submitted to preparative HPLC using a C18 column (21.2 × 250 mm, 100 Å, 5 µm particle size, Kromasil®), an isocratic flow MeOH:H2O 1:1. Injections were performed with a 100 µl loop. The flow rate was 10 ml/min for RBE1, RBE2 and 6 ml/min for HR1.
Parasites
Leishmania amazonensis parasites (MHOM/BR88/BA-125) were isolated from infected C57BL/6 mice lesions and cultivated at 26 ºC on NNN (Novy, McNeal, Nicolle) medium for 15 days. The promastigote forms were isolated and maintained at 26 ºC in Warren's medium (brain–heart infusion 37 g/l plus haemin 10 mg/l and folic acid 10 mg/l) supplemented with 10% fetal bovine serum.
Trypanosoma cruzi epimastigote forms (strain Y) were maintained at 26 ºC in LIT (liver infusion tryptose) medium, supplemented with 10% fetal calf serum. For both strains the cell population was monitored and the transplantation was made when they reached the growth stationary phase.
Antileishmanial and antitrypanosomal susceptibility test
Leishmania amazonensis promastigotes (5 × 105 parasites/ml) and T. cruzi (5 × 105 parasites/ml) were inoculated in a 24-well plate containing Warren's medium supplemented with 10% inactivated FBS with different concentrations (0.75–200 µM, obtained by three serial dilutions in DMSO) of the tested compounds (1, 6, 9 or 12).
The final volume was 2 ml/well, with a final concentration of DMSO less than 0.1%. The plates were incubated 26 ºC for 72 h. The cells were fixed with 3.8% paraformaldehyde and the density was determined using a Neubauer counting chamber.
All the experiments were realized in triplicate. The antileishmanial and anti-trypanosomal activities were expressed as the percentage of growth inhibition in compound tested cells and IC50 values were calculated.
Results and discussion
The preparative HPLC separation performed on the RBE1 and RBE2 extracts allowed the isolation of eight fractions (F2-F5 and H2-H5, respectively, Fig. 1). All the fractions were analyzed by HPLC/MS and NMR spectroscopy, allowing the identification of eight compounds.
Analytical and preparative HPLC chromatograms for RBE1 and RBE2 fractions. Analytical conditions: C18 column (4.6 × 250 mm, 100 Å, 5 µm particle size, Kromasil®), guard column (4.6 × 20 mm, 5 µm particle size), isocratic flow of MeOH:H2O (1:1) at a rate of 0.6 ml/min, injection volume of 20 µl. Preparative conditions: C18 column (21.2 × 250 mm, 5 µm, 100 Å), an isocratic flow MeOH:H2O 1:1 and injection volume of 100 µl. The flow rate was 10 ml/min, DAD254nm.
Pure 5,7,8-trimethoxycoumarin (1) (7 mg) was obtained from F2. Sabandin (2) and cubreuva lactone (3) eluted together (13.3 mg) in F3. A mixture of isopimpinelin (4) and 5,7-dimethoxycoumarin (5) (8.6 mg) was recovered from F4. Braylin (6) (51.10 mg) was purified from F5.
The chromatographic analysis of the extracts obtained from RBE2 allowed the identification of compounds 2 and 3 in fraction H2; 4 and 5 in fraction H3; 6 in fraction H4. Pteleine (7) could not be separated from maculine (8) (5.6 mg) in fraction H5.
The preparative scale HPLC analysis of fraction HR1 (Fig. 2) allowed the isolation of pure syringaresinol (9) (R1, 20.9 mg), a mixture of skimianine (10) and robustine (11) (R5, 3.9 mg), pure y-fagarine (12) (R6, 8.7 mg) and dictamine (13) (R7, 10 mg). Other peaks were observed on the HPLC chromatograms, but the amounts of these materials were too small and did not allow the identification of the compounds.
Analytical and preparative HPLC chromatograms for HR1 fraction. Analytical conditions: C18 column (4.6 × 250 mm, 100 Å, 5 µm particle size, Kromasil®), guard column (4.6 × 20 mm, 5 µm particle size), isocratic flow of MeOH:H2O (1:1) at a rate of 0.6 ml/min, injection volume of 20 µl. Preparative conditions: C18 column (21.2 × 250 mm, 5 µm, 100 Å), an isocratic flow MeOH:H2O 1:1, injection volume of 100 µl at flow 6 ml/min, DAD254nm.
The identification of the chemical structures of the compounds was based on the comparison of the spectroscopic data of the isolated fractions to literature data (Pusset et al., 1991Pusset, J., Lopez, J.L., Pais, M., Neirabeyeh, M.A., Veillon, J.-M., 1991. Isolation and 2D NMR studies of alkaloids from Comptonella sessilifoliola. Planta Med. 57, 153-155.; Cuca et al., 1998Cuca, L.E., Martinez, J.C., Delle Monache, F., 1998. Alcaloides presentes em Hortia colombiana. Rev. Colomb. Quim. 27, 23-29.; Chakravarty et al., 1999Chakravarty, A.K., Sarkar, T., Masuda, K., Shiojima, K., 1999. Carbazole alkaloids from roots of Glycosmis arborea. Phytochemistry 50, 1263-1266.; Leong et al., 1999Leong, Y.-W., Harrison, L.J., Powell, A.D., 1999. Phenanthrene and other aromatic constituents of Bulbophyllum vaginatum. Phytochemistry 50, 1237-1241.; Yoo et al., 2002Yoo, S., Kim, J., Kang, S., Son, K., Chang, H., Kim, H., Bae, K., Lee, C.-O., 2002. Constituents of the fruits and leaves of Euodia daniellii. Arch. Pharm. Res. 25, 824-830.; Bhoga et al., 2004Bhoga, U., Mali, R.S., Adapa, S.R., 2004. New synthesis of linear furoquinoline alkaloids. Tetrahedron Lett. 45, 9483-9485.; Randrianarivelojosia et al., 2005Randrianarivelojosia, M., Mulholland, D.A., Mc Farland, K., 2005. Prenylated coumarins from Cedrelopsis longibracteata (Ptaeroxylaceae). Biochem. Syst. Ecol. 33, 301-304.; Maes et al., 2005Maes, D., Vervisch, S., Debenedetti, S., Davio, C., Mangelinckx, S., Giubellina, N., De Kimpe, N., 2005. Synthesis and structural revision of naturally occurring ayapin derivatives. Tetrahedron 61, 2505-2511., 2008Maes, D., Vervisch, S., Debenedetti, S., Davio, C., Mangelinckx, S., Giubellina, N., De Kimpe, N., 2005. Synthesis and structural revision of naturally occurring ayapin derivatives. Tetrahedron 61, 2505-2511.; Nunes et al., 2005Nunes, F.M., Barros-Filho, B.A., de Oliveira, M.C.F., Andrade-Neto, M., de Mattos, M.C., Mafezoli, J., Pirani, J.R., 2005. 1H and 13C NMR spectra of 3,8-dimethoxyfuro[3,2-g]coumarin and maculine from Esenbeckia grandiflora Martius (Rutaceae). Magn. Reson. Chem. 43, 864-866.; Chlouchi et al., 2006Chlouchi, A., Girard, C., Tillequin, F., Bévalot, F., Waterman, P.G., Muyard, F., 2006. Coumarins and furoquinoline alkaloids from Philotheca deserti var. deserti (Rutaceae). Biochem. Syst. Ecol. 34, 71-74.; Kutubi et al., 2011Kutubi, M.S., Hashimoto, T., Kitamura, T., 2011. Improved synthesis of coumarins by iron(III)-catalyzed cascade reaction of propiolic acids and phenols. Synthesis, http://dx.doi.org/10.1055/s-0030-1258473.
http://dx.doi.org/10.1055/s-0030-1258473...
).
The 1H NMR spectrum of compound 1 show three singlets at 3.91, 3.92 and 3.97 ppm, characteristic shifts of methoxy groups on an aromatic ring, and a single aromatic hydrogen at 6.17 ppm. Two signals at 6.17 and 7.98 ppm indicate the presence of a conjugated double bond. The signal of the lactone carbonyl group is shown on the 13C NMR at 160.8 ppm. The structure of 5,7,8-trimethoxycoumarin (1) was confirmed by comparison with literature data (Maes et al., 2008Maes, D., Riveiro, M.E., Shayo, C., Davio, C., Debenedetti, S., De Kimpe, N., 2008. Total synthesis of naturally occurring 5,6,7- and 5,7,8-trioxygenated coumarins. Tetrahedron 64, 4438-4443.). The analytical HPLC analysis of fractions F3 and H2 showed similar retention times, suggesting a similar composition. The analysis of the 1H NMR spectra of F2 reveals the presence of protons with characteristic shifts found in coumarins. Signals at 4.12, 4.02 and 3.99 ppm suggest the presence of a dimethoxy and a methoxy coumarin with a 5:1 ratio. Only one aromatic proton is present on the spectra (6.48 ppm), which was assigned to the minor compound, a methoxy coumarin. Signals at 5.99 and 6.01 ppm were attributed to methylenedioxy protons present in both structures. Duplicated signals are also observed on the 13C NMR spectra. The comparison with literature data (Maes et al., 2005Maes, D., Vervisch, S., Debenedetti, S., Davio, C., Mangelinckx, S., Giubellina, N., De Kimpe, N., 2005. Synthesis and structural revision of naturally occurring ayapin derivatives. Tetrahedron 61, 2505-2511.) confirmed the structures of coumarins cubreuva lactone 2 (minor compound) and sabandine 3 (major compound). The chromatograms of F4 and H3 fractions show two overlapping peaks in analytical chromatogram (t
R = 33.3 mn and 39.7 mn) which could not be separated in these preparative conditions. The 1H NMR analysis of F4 reveals the presence of two dimethoxycoumarins, with a 1:2 ratio. Duplets at 7.0 ppm and 7.63 ppm (minor product) and at 6.28 and 6.41 ppm were attributed to aromatic or furanic protons. The comparison of the 13C NMR data with literature (Yoo et al., 2002Yoo, S., Kim, J., Kang, S., Son, K., Chang, H., Kim, H., Bae, K., Lee, C.-O., 2002. Constituents of the fruits and leaves of Euodia daniellii. Arch. Pharm. Res. 25, 824-830.; Kutubi et al., 2011Kutubi, M.S., Hashimoto, T., Kitamura, T., 2011. Improved synthesis of coumarins by iron(III)-catalyzed cascade reaction of propiolic acids and phenols. Synthesis, http://dx.doi.org/10.1055/s-0030-1258473.
http://dx.doi.org/10.1055/s-0030-1258473...
) along with the MS spectra, allowed the identification of 5,7-dimethoxycoumarin 4 ([M+H]+ = 207,06779 Da) and isopimpinellin 5 ([M+H]+ = 247,06251 Da). The chromatograms of F5 and H4 show the presence of one compound ([M+Na]+ = 281,08164). The 1H NMR spectra shows the characteristic signals of the benzopyrone nucleus of the coumarins, carrying one methoxygroup. One peak at 1.52 ppm was attributed to two methyl groups belonging to a 2,2-dimethylpyrane cycle. The structure of braylin (6) was confirmed by comparison with literature data (Randrianarivelojosia et al., 2005Pusset, J., Lopez, J.L., Pais, M., Neirabeyeh, M.A., Veillon, J.-M., 1991. Isolation and 2D NMR studies of alkaloids from Comptonella sessilifoliola. Planta Med. 57, 153-155.). A mixture of compounds 7 and 8 was isolated from the H5 fraction. The analysis of 1H and 13C NMR spectra is consistent with methoxylated furoquinoline alkaloids. The chemical structures were established by comparison with literature data as maculine (7) (Nunes et al., 2005Nunes, F.M., Barros-Filho, B.A., de Oliveira, M.C.F., Andrade-Neto, M., de Mattos, M.C., Mafezoli, J., Pirani, J.R., 2005. 1H and 13C NMR spectra of 3,8-dimethoxyfuro[3,2-g]coumarin and maculine from Esenbeckia grandiflora Martius (Rutaceae). Magn. Reson. Chem. 43, 864-866.) and pteleine (8) (Pusset et al., 1991Patiño, L.O.J., Prieto, R.J.A.L., Cuca, S.L.E., 2012. Zanthoxylum genus as potential source of bioactive compounds. In: Bioactive Compounds in Phytomedicine. InTech, P.I.R.E.). Pure (+)-seringaresinol (9) was isolated from the R1 fraction and identified by comparison with published data (Leong et al., 1999Leong, Y.-W., Harrison, L.J., Powell, A.D., 1999. Phenanthrene and other aromatic constituents of Bulbophyllum vaginatum. Phytochemistry 50, 1237-1241.). Compounds 10 and 11 could not be separated from fraction R5 and were isolated as a mixture (31:69 ratio). The NMR spectra revealed characteristic signals assigned to furoquinoline alkaloids. The compounds were identified as skimianine (10) (Chakravarty et al., 1999Chakravarty, A.K., Sarkar, T., Masuda, K., Shiojima, K., 1999. Carbazole alkaloids from roots of Glycosmis arborea. Phytochemistry 50, 1263-1266.) and robustine (11) (Chlouchi et al., 2006Chlouchi, A., Girard, C., Tillequin, F., Bévalot, F., Waterman, P.G., Muyard, F., 2006. Coumarins and furoquinoline alkaloids from Philotheca deserti var. deserti (Rutaceae). Biochem. Syst. Ecol. 34, 71-74.). Finally, y-fagarine (12) and dictamine (13) were obtained as pure compounds in fraction R6 and R7, respectively, and comparison with published data (Bhoga et al., 2004Bhoga, U., Mali, R.S., Adapa, S.R., 2004. New synthesis of linear furoquinoline alkaloids. Tetrahedron Lett. 45, 9483-9485.; Cuca et al., 1998Cuca, L.E., Martinez, J.C., Delle Monache, F., 1998. Alcaloides presentes em Hortia colombiana. Rev. Colomb. Quim. 27, 23-29.) allowed the confirmation of their chemical structures.
Coumarins, lignans and alkaloids are commonly found in plants belonging to the Rutaceae family. The group of alkaloid most frequently reported in the genus Zanthoxylum is isoquinoline, mainly benzophenanthridines (Fish and Waterman, 1973Fish, F., Waterman, P.G., 1973. Chemosystematics in the Rutaceae II. The chemosystematics of the Zanthoxylum/Fagara complex. Taxon 22, 177-203.; Hohlemwerger et al., 2012Hohlemwerger, S.V.A., Sales, E.M., Costa, R.S., Velozo, E.S., Guedes, M.L.S., 2012. Alcaloides das cascas das raízes de Zanthoxylum spp.. Quim. Nova 35, 2173-2176.). Previous chemical studies of Z. tingoassuiba have shown the presence of this class of compounds, along with aporphine and protoberberine alkaloids, as well as a N-methylanthranilate derivative (Silva et al., 2008Silva, C.V., Detoni, C.B., Velozo, E.S., Guedes, M.L.S., 2008. Alcalóides e outros metabólitos do caule e frutos de Zanthoxylum tingoassuiba A St. Hil.. Quim. Nova 31, 2071-2075.; Hohlemwerger et al., 2012Hohlemwerger, S.V.A., Sales, E.M., Costa, R.S., Velozo, E.S., Guedes, M.L.S., 2012. Alcaloides das cascas das raízes de Zanthoxylum spp.. Quim. Nova 35, 2173-2176.; Costa et al., 2017Costa, R.S., Lins, M.O., Le Hyaric, M., Barros, T.F., Velozo, E.S., 2017. In vitro antibacterial effects of Zanthoxylum tingoassuiba bark root extracts and two of its alkaloids against multiresistant Staphylococcus aureus. Rev. Bras. Farmacogn. 27, 195-198.). Furanocoumarins (7-oxygenated coumarins) are frequently found in the Rutaceae family and recently three new substances have been identified in Z. avicennae (Chen et al., 2015Chen, J.-J., Yang, C.-K., Kuo, Y.-H., Hwang, T.-L., Kuo, W.-L., Lim, Y.-P., Sung, P.-J., Chang, T.-H., Cheng, M.-J., 2015. New coumarin derivatives and other constituents from the stem bark of Zanthoxylum avicennae: effects on neutrophil pro-inflammatory responses. Int. J. Mol. Sci. 16, 9719-9731.). This report describes for the first time the presence of five coumarins in Z. tingoassuiba: 5,7,8-trimethoxycoumarin (1), sabandin (2), cubreuva lactone (3), 5,7-dimethoxycoumarin (5) and braylin (6).
The present work also reports for the first time the occurrence of furoquinoline alkaloids in Z. tingoassuiba species. Seven different chemical structures were identified: isopimpinelin (4), pteleine (7), maculine (8), skimianine (10), robustine (11), y-fagarine (12) and dictamine (13). Additionally, syringaresinol (9) a furofuran type lignan is isolated for the first time from Z. tingoassuiba in the present study.
Spectral data
5,7,8-trimethoxycoumarin (1): (500 MHz. CDCl3): 6.16 (1, d, J = 9.7, H3), 7.98 (1H, d, J = 9.7, H4), 6.35 (1H, s, H6), 3.91 (3H, s, –OCH3), 3.92 (3H, s, –OCH3), 3.97 (3H, s, –OCH3). [M+H]+: 237.07917 Da, C12H12O5. UV λ max: 260.26 nm; 323.38 nm.
Sabandin (2): (500 MHz, CDCl3): 6.21 (1H, d, J = 10.0 H3), 7.92 (1H, d, J = 10.0 H4), 6.02 (2H, s, –OCH2O–), 4.02 (3H, s, –OCH3), 4.05 (3H, s, –OCH3). [M+H]+: 251.005834 Da, C12H10O6. UV λ max: 239.25 nm; 327.15 nm.
Cubreuva lactone (3): (500 MHz, CDCl3): 6.19 (1H, d, J = 9.6 H3), 7.93 (1H, d, J = 9.5 H4), 6.49 (1H, s, H8), 6.00 (2H, s, –OCH2O–), 4.12 (3H, s, –OCH3). [M+H]+: 221.04723 Da, C11H8O5.
Isopimpinelin (4): (500 MHz, CDCl3): 6.30 (1H, d, J = 9.8 H3), 8.13 (1H, d, J = 9.8 H4), 7.63 (1H, d, J = 2.3 H2′), 7.00 (1H, d, J = 2.3 H3′), 4.16 (3H, s, –OCH3), 4.17 (3H, s, –OCH3). [M+H]+: 247.06251 Da, C13H10O5. UV λ max: 221.75 nm; 267.34 nm; 313.09 nm.
5,7-dimethoxycoumarin (5): (500 MHz, CDCl3): 6.16 (1H, d, J = 9.7 H3), 7.97 (1H, d, J = 9.7 H4), 6.29 (1H,d, J = 2.1 H6), 6.42 (1H, d, J = 2.1 H8), 3.89 (3H, s, –OCH3), 3.86 (3H, s, –OCH3). [M+H]+: 207.06779 Da, C11H10O4. UV λ max: 250.51 nm; 327.67 nm.
Braylin (6): (500 MHz, CDCl3): 6.26 (1H, d, J = 9.5 H3), 7.59 (1H, d, J = 9.5 H4), 6.80 (1H, s, H6), 6.89 (1H, d, J = 10.05 H1′), 5.76 (1H, d, J = 10.05 H2′), 1.53 (6H, s, –CH3 4′ and 5′), 3.89 (3H, s, –OCH3). [M+H]+: 259.09914 Da, C15H14O4. UV λ max: 225.63 nm; 350.81 nm.
Pteleine (7): (500 MHz, CDCl3): 8.07 (1H, d, J = 9.2 H5), 7.40 (1H, dd, J = 9.2, J = 2.9 H6), 7.55 (1H, d, J = 2.9 H8), 7.66 (1H, d, J = 2.7 H2′), 7.11 (1H, d, J = 2.7 H3′), 3.96 (3H, s, –OCH3), 4.50 (3H, s, –OCH3). [M+H]+: 230.08254 Da, C13H11NO3. UV λ max: 236.78 nm; 306.71 nm.
Maculine (8): (500 MHz, CDCl3): 7.53 (1H, s H5), 7.51 (1H, s H8), 7.61 (1H, d, J = 2.7 H2′), 7.08 (1H, d, J = 2.7 H3′), 6.00 (2H, s, –OCH2O-), 4.46 (3H, s, –OCH3). [M+H]+: 244.06135 Da, C13H9NO4. UV λ max: 243.70 nm; 307.20 nm.
Syringaresinol (9): (500 MHz, CDCl3): 3.10 (1H, m, H1 and H5), 4.74 (2H, d, J = 4.1 H2 and H6), 4.29 (2H, dd, J = 6.8 and 9,1 H4eq and H8eq), 3.91 (2H, m, H4ax and H8ax), 6.59 (4H, s, H2′, 2″ and H6′, 6″), 3.90 (12H, –OCH3), 5.51 (2H, sl –OH). [M+H]+: 419.17267 Da, C22H26O8. UV λ max: 206.29 nm.
Skimianine (10): (400 MHz, CDCl3): 8.04 (1H, d, J = 9.4 H5), 7.26 (1H, d, J = 9.4 H6), 7.59 (1H, d, J = 2.8 H2′), 7.06 (1H, d, J = 2.8 H3′), 4.04 (3H, s, –OCH3), 4.11 (3H, s, –OCH3) 4,46 (3H, s, –OCH3). [M+H]+: 260.09963 Da, C14H13NO4. UV λ max: 248.02 nm; 320.99 nm
Robustine (11): (400 MHz, CDCl3): 7.75 (1H, dd, J = 8.6, J = 1.2 H5), 7.35 (1H, dd, J = 7.5, J = 7.5 H6), 7.20 (1H, dd, J = 7.5 J = 1,2 H7), 7.65 (1H, d, J = 2.8 H2′), 7.13 (1H, d, J = 2.8 H3′), 4.47 (3H, s, –OCH3). [M+H]+: 216.06782 Da, C12H9NO3. UV λ max: 243.76 nm; 311.01 nm.
γ-Fagarine (12): (500 MHz, CDCl3): 7.87 (1H, dd, J = 8.6 and 1.0 H5), 7.37 (1H, dd, J = 7.7 and 8.6 H6), 7,08 (1H, dd, J = 7.7 and 1.0 H7), 7.67 (1H, d, J = 2.8 H2′), 7.10 (1H, d, J = 2.8 H3′), 4,10 (3H, s, –OCH3), 4.47 (3H, s, –OCH3). [M+H]+: 230.08335 Da, C13H11NO3. UV λ max: 243.09 nm; 310.46 nm.
Dictamine (13): (300 MHz, CDCl3): 8.28 (1H, ddd, J = 8.5, 1.5, 0.6 H5), 7.45 (1H, ddd, J = 8.1, 8.1, 1.2 H6), 7.69 (1H, ddd, J = 8.4, 8.5, 1.5 H7), 8.02 (1H, ddd, J = 8.5, 1.2, 0.3 H8), 7.63 (1H, d, J = 2.8H2′), 7.08 (1H, d, J = 2.8 H3′), 4.44 (3H, s, –OCH3). [M+H]+: 200.07320 Da, C12H9NO2. UV λ max: 236.18 nm; 309.71 nm.
Antiparasitic activity
Pure compounds 1, 6, 9, 12 were tested against epimastigote forms of T. cruzi, Y-strain. The results are detailed in Fig. 3 and Table 1.
Proliferation of Trypanosoma cruzi treated with 5,7,8-trimethoxycoumarin (1), braylin (6), syringaresinol (9) and γ-fagarine (12). Epimastigotes (5 × 105 cells/ml) were incubated in different concentrations of the tested compounds at 26 ºC for 72 h and cell densities were determined by light microscopy. 20 µM benznidazole was employed as positive control. Statistical analysis was performed using One-way ANOVA and Tukey pos-test (*** p < 0.001, ** p < 0.01, * p < 0.05).
Compounds 6, 9 and 12 significantly reduced the proliferation of the parasite at low concentrations (6.2, 1.2 and 0.75 µM, respectively). The highest levels of inhibition were obtained at concentrations of 100 µM (γ-fagarine, 77%), 78 µM (syringaresinol, 80%), 200 µM (braylin 75% respectively) and 100 µM (5,7,8-trimethoxycoumarin, 75%). The most active substances were syringaresinol 9 (IC50 = 7.55 µM) and 5,7,8-trimethoxycoumarin 1 (IC50 = 25.5 µM), while γ-fagarine (12) and braylin (6) displayed moderate activities (IC50 = 33.35 and 59.8 µM, respectively).
The four compounds also demonstrated a significant capacity to inhibit the growth of promastigote forms of L. amazonensis (Fig. 4) at concentrations of 78 µM (syringaresinol, 98%), 100 µM (γ-fagarine, 100%) and 5,7,8-trimethoxycoumarin 91%), and 200 µM (braylin 100%). At lower concentrations, 5,7,8-trimethoxycoumarin 1, braylin 6 and γ-fagarine 12 were more active than the positive control. Braylin and 5,7,8-trimethoxycoumarin stimulated the proliferation of the parasite at the concentration of 12.5 µM.
Proliferation of Leishmania amazonensis treated with 5,7,8-trimethoxycoumarin (1), braylin (6), syringaresinol (9) and γ-fagarine (12). Promastigotes (5 × 105 cells/ml) were incubated in different concentrations of the tested compounds at 26 ºC for 72 h and cell densities were determined by light microscopy. Amphotericin (5 µM) was employed as positive control. Statistical analysis was performed using One-way ANOVA and Tukey pos-test (*** p < 0.001, ** p < 0.01, * p < 0.05).
The most active substances were syringaresinol (9) (IC50 12.0 µM) and γ-fagarine (12) (IC50 31.3 µM) followed by 5,7,8-trimethoxycoumarin (1) (IC50 57.7 µM) and braylin (6) (IC50 70.0 µM).
Interestingly the antiparasitic activity of the natural products approached here displayed effectivity levels similar to the positive controls benznidazole and amphotericin B, used for trypanocidal and leishmanicidal activities, respectively.
Coumarins are known for their antitrypanosomal (Guíñez et al., 2013Guíñez, R.F., Matos, M.J., Vazquez-Rodriguez, S., Santana, L., Uriarte, E., Olea-Azar, C., Maya, J.D., 2013. Synthesis and evaluation of antioxidant and trypanocidal properties of a selected series of coumarin derivatives. Future Med. Chem. 5, 1911-1922.; Chen et al., 2015Chen, J.-J., Yang, C.-K., Kuo, Y.-H., Hwang, T.-L., Kuo, W.-L., Lim, Y.-P., Sung, P.-J., Chang, T.-H., Cheng, M.-J., 2015. New coumarin derivatives and other constituents from the stem bark of Zanthoxylum avicennae: effects on neutrophil pro-inflammatory responses. Int. J. Mol. Sci. 16, 9719-9731.) and antileishmanial activities (Reyes-Chilpa et al., 2008Reyes-Chilpa, R., Estrada-Muñiz, E., Vega-Avila, E., Abe, F., Kinjo, J., Hernández-Ortega, S., 2008. Trypanocidal constituents in plants: 7 Mammea-type coumarins. Mem. Inst. Oswaldo Cruz 103, 431-436.; Mandlik et al., 2016Mandlik, V., Patil, S., Bopanna, R., Basu, S., Singh, S., 2016. Biological activity of coumarin derivatives as anti-leishmanial agents. PLOS ONE 11, e0164585.). Previous studies have shown that they act on an enzyme glyceraldehyde-3-phosphate dehydrogenase (GAPDH) of the glycolytic pathway of the trypanosomatids (Freitas et al., 2009Freitas, R.F., Prokopczyk, I.M., Zottis, A., Oliva, G., Andricopulo, A.D., Trevisan, M.T.S., Vilegas, W., Silva, M.G.V., Montanari, C.A., 2009. Discovery of novel Trypanosoma cruzi glyceraldehyde-3-phosphate dehydrogenase inhibitors. Bioorg. Med. Chem. 17, 2476-2482.) and that they inhibit the growth of L. amazonensis and altered the parasite structure (Brenzan et al., 2007Brenzan, M.A., Nakamura, C.V., Dias Filho, B.P., Ueda-Nakamura, T., Young, M.C.M., Cortez, D.A.G., 2007. Antileishmanial activity of crude extract and coumarin from Calophyllum brasiliense leaves against Leishmania amazonensis. Parasitol. Res. 101, 715-722.). A survey of the literature showed that there is no report on the antitrypanosomal activities of the coumarins 1 (5,7,8-trimethoxycoumarin) and 6 (braylin) isolated in this work. The lignan (+)-syringaresinol 9 was the most active of the tested compounds against both parasites. Recently a study conducted by de Sousa et al. (2014)de Sousa, L.R.F., Ramalho, S.D., Burger, M.C., Nebo, D.M., Fernandes, L., da Silva, J.B., Iemma, M.F.G.F., Corrêa, M.R.C., Souza, C.J., Lima, D.H.F., Vieira, M.I.S.P.C., 2014. Isolation of arginase inhibitors from the bioactivity-guided fractionation of Byrsonima coccolobifolia leaves and stems. J. Nat. Prod. 77, 392-396. showed that syringaresinol is able to inhibit arginase from L. amazonensis a key enzyme involved in the polyamine synthesis and in the production of nitric oxide, and its ability to inhibit T. b. brucei growth has been described Alamzeb et al. (2013)Alamzeb, M., Khan, M.R., Ali, S., Mamoon-Ur-Rashid Khan, A.A., 2013. Bioassay guided isolation and characterization of anti-microbial and anti-trypanosomal agents from Berberis glaucocarpa Stapf. Afr. J. Pharm. Pharmacol. 7, 2065-2071.. Inhibition of L. amazonensis arginase by Cecropia pachystachya flavonoids present antiparasitic activity associated to mitochondrial and kinetoplast DNA disorganization (Cruz et al., 2013Cruz, E.M., da Silva, E.R., Maquiaveli, C.C., Alves, E.S.S., Lucon, J.F., Reis, M.B.G., Toledo, C.E.M., Cruz, F.G., Vannier-Santos, M.A., 2013. Leishmanicidal activity of Cecropia pachystachya flavonoids: arginase inhibition and altered mitochondrial DNA arrangement. Phytochemistry 89, 71-77.). Previous studies have shown that lignans isolated from Z. naranjillo showed high trypanocidal effect against the trypomastigote forms of T. cruzi (Bastos et al., 1999Bastos, J.K., Albuquerque, S., Silva, M.L., 1999. Evaluation of the trypanocidal activity of lignans isolated from the leaves of Zanthoxylum naranjillo. Planta Med. 65, 541-544.). De Souza et al. (2005)de Souza, V.A., da Silva, R., Pereira, A.C., Royo, V.A., Saraiva, J., Montanheiro, M., de Souza, G.H.B., da Silva Filho, A.A., Grando, M.D., Donate, P.M., Bastos, J.K., Albuquerque, S., Silva, M.L.A., 2005. Trypanocidal activity of (-)-cubebin derivatives against free amastigote forms of Trypanosoma cruzi. Bioorg. Med. Chem. Lett. 15, 303-307. demonstrated the activity of lignan derivatives against free amastigote forms of Trypanosoma cruzi, No report was found on the activity of (+)-syringaresinol 9 against T. cruzi or L. amazonensis. It is noteworthy that, under the conditions employed here, this bioagent was more trypanocidal than benznidazole, used as positive control.
The comparison of the IC50 values obtained for both parasites show that γ-fagarine had about the same potency against L. amazonensis and T. cruzi (IC50 31.34 and 33.35 µM, respectively), while the other tested compounds were more active against T. cruzi. In a study performed by Ferreira et al. (2010)Ferreira, M.E., Arias, A.R., Yaluff, G., de Bilbao, N.V., Nakayama, H., Torres, S., Schinini, A., Guy, I., Heinzen, H., Fournet, A., 2010. Antileishmanial activity of furoquinolines and coumarins from Helietta apiculata. Phytomedicine 17, 375-378. the alkaloid γ-fagarine (12) demonstrated antiparasitic activity against promastigot forms of L. amazonensis (IC50 17.3 µM), L. infantum and L. braziliensis (26.5 µM and 22.2 µM, respectively). A significant in vitro inhibitory activity against the promastigotes of L. tropica (IC50 0.37 µM) was also shown by γ-fagarine (Östan et al., 2007Östan, I., Saglam, H., Limoncu, M.E., Ertabaklar, H., Toz, S.Ö., Özbel, Y., Özbilgin, A., 2007. In vitro and in vivo activities of Haplophyllum myrtifolium against Leishmania tropica. New Microbiol. 30, 439-445.).
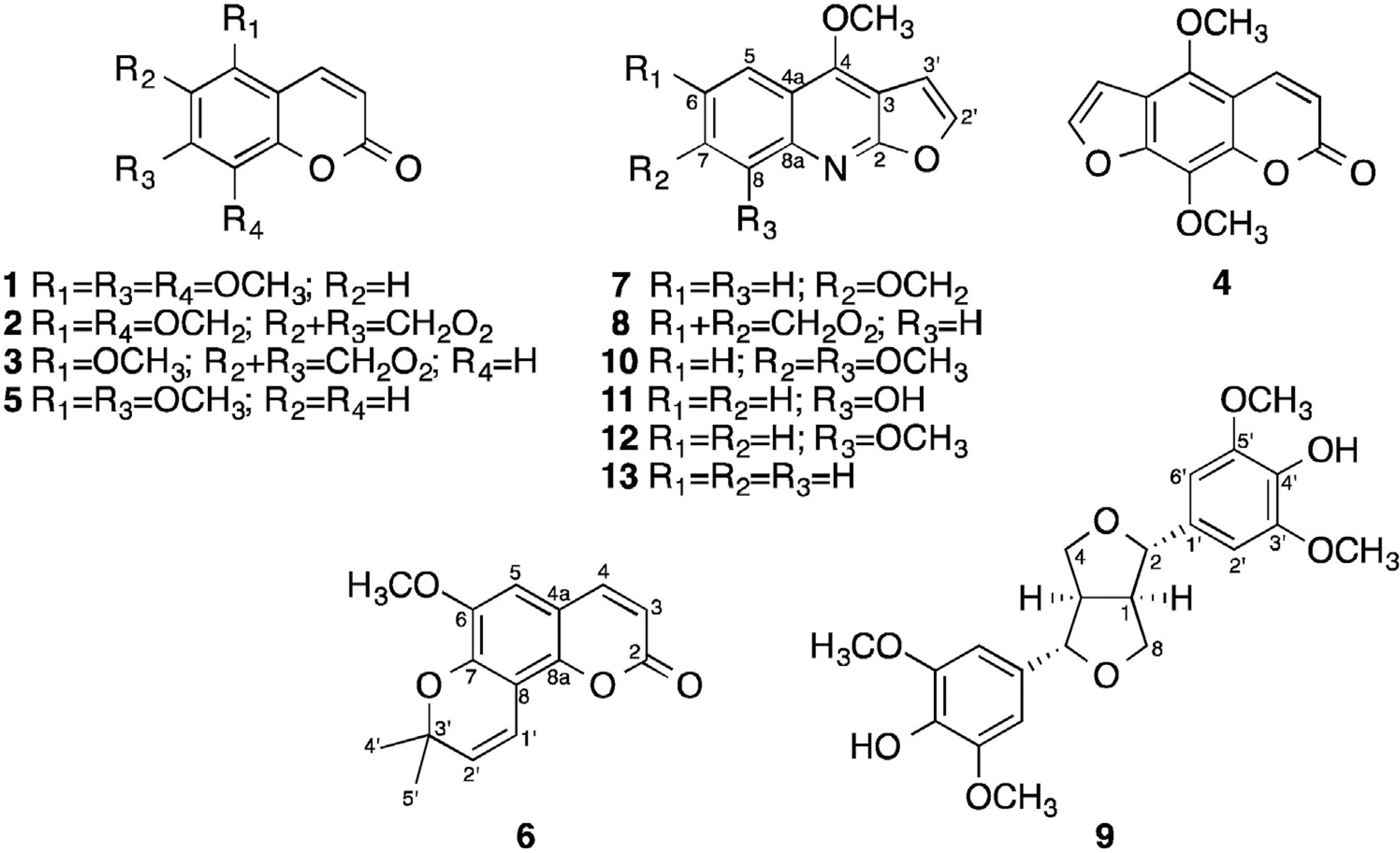
Conclusion
The phytochemical study of the roots from Zanthoxylum tingoassuiba allowed the identification of six coumarins, six furoquinoline alkaloids and a furofuranic lignan. Eleven of these compounds were isolated for the fist time in this species (isopimpeneline and braylin have been previously described). The results obtained in the in vitro test against T. cruzi and L. Amazonensis showed that some of the compounds found in the species display antiparisitic activity. These findings contribute to a better understanding of the chemical composition of this species of occurrence in the Brazilian semi-arid region and to the knowledge of the Brazilian biodiversity as a source for drug discovery.
Ethical disclosures
-
Protection of human and animal subjects. The authors declare that no experiments were performed on humans or animals for this study.
-
Confidentiality of data. The authors declare that no patient data appear in this article.
-
Right to privacy and informed consent. The authors declare that no patient data appear in this article.
-
1
Present address: Laboratório de Toxoplasmose e outras Protozooses, Instituto Oswaldo Cruz, Fundação Oswaldo Cruz; Fiocruz/RJ, Brazil.
Acknowledgments
We thank Prof. Norberto Peporine Lopes (Faculty of Pharmaceutical Sciences of Ribeirão Preto, University of São Paulo) for the availability of laboratory and supplies. The authors thank CAPES and CNPq for the grants.
References
- Alamzeb, M., Khan, M.R., Ali, S., Mamoon-Ur-Rashid Khan, A.A., 2013. Bioassay guided isolation and characterization of anti-microbial and anti-trypanosomal agents from Berberis glaucocarpa Stapf. Afr. J. Pharm. Pharmacol. 7, 2065-2071.
- Annang, F., Genilloud, O., Vicente, F., 2016. Contribution of natural products to drug discovery. In: Tropical Diseases, Comprehensive Analysis of Parasite Biology: From Metabolism to Drug Discovery. Wiley-VCH Verlag GmbH and Co., KGaA, pp. 75–104.
- Bastos, J.K., Albuquerque, S., Silva, M.L., 1999. Evaluation of the trypanocidal activity of lignans isolated from the leaves of Zanthoxylum naranjillo Planta Med. 65, 541-544.
- Bhoga, U., Mali, R.S., Adapa, S.R., 2004. New synthesis of linear furoquinoline alkaloids. Tetrahedron Lett. 45, 9483-9485.
- Bou, D.D., Tempone, A.G., Pinto, E.G., Lago, J.H.G., Sartorelli, P., 2014. Antiparasitic activity and effect of casearins isolated from Casearia sylvestris on Leishmania and Trypanosoma cruzi plasma membrane. Phytomedicine 21, 676-681.
- Braga, F.G., Bouzada, M.L.M., Fabri, R.L., Matos, M.O., Moreira, F.O., Scio, E., Coimbra, E.S., 2007. Antileishmanial and antifungal activity of plants used in traditional medicine in Brazil. J. Ethnopharmacol. 111, 396-402.
- Brenzan, M.A., Nakamura, C.V., Dias Filho, B.P., Ueda-Nakamura, T., Young, M.C.M., Cortez, D.A.G., 2007. Antileishmanial activity of crude extract and coumarin from Calophyllum brasiliense leaves against Leishmania amazonensis Parasitol. Res. 101, 715-722.
- Cechinel Filho, V., Meyre-Silva, C., Niero, R., Mariano, L.N.B., Nascimento, F.G., Farias, I.V., Gazoni, V.F., dos Santos Silva, B., Gimenez, A., Gutierrez-Yapu, D., Salamanca, E., Malheiros, A., 2013. Evaluation of antileishmanial activity of selected Brazilian plants and identification of the active principles. Evid. Based Complement Altern. Med., http://dx.doi.org/10.1155/2013/265025
» http://dx.doi.org/10.1155/2013/265025 - Chakravarty, A.K., Sarkar, T., Masuda, K., Shiojima, K., 1999. Carbazole alkaloids from roots of Glycosmis arborea Phytochemistry 50, 1263-1266.
- Chen, J.-J., Yang, C.-K., Kuo, Y.-H., Hwang, T.-L., Kuo, W.-L., Lim, Y.-P., Sung, P.-J., Chang, T.-H., Cheng, M.-J., 2015. New coumarin derivatives and other constituents from the stem bark of Zanthoxylum avicennae: effects on neutrophil pro-inflammatory responses. Int. J. Mol. Sci. 16, 9719-9731.
- Chlouchi, A., Girard, C., Tillequin, F., Bévalot, F., Waterman, P.G., Muyard, F., 2006. Coumarins and furoquinoline alkaloids from Philotheca deserti var. deserti (Rutaceae). Biochem. Syst. Ecol. 34, 71-74.
- Costa, R.S., Lins, M.O., Le Hyaric, M., Barros, T.F., Velozo, E.S., 2017. In vitro antibacterial effects of Zanthoxylum tingoassuiba bark root extracts and two of its alkaloids against multiresistant Staphylococcus aureus Rev. Bras. Farmacogn. 27, 195-198.
- Cragg, G.M., Newman, D.J., 2013. Natural products: a continuing source of novel drug leads. Biochim. Biophys. Acta Gen. Subj. 1830, 3670-3695.
- Cruz, E.M., da Silva, E.R., Maquiaveli, C.C., Alves, E.S.S., Lucon, J.F., Reis, M.B.G., Toledo, C.E.M., Cruz, F.G., Vannier-Santos, M.A., 2013. Leishmanicidal activity of Cecropia pachystachya flavonoids: arginase inhibition and altered mitochondrial DNA arrangement. Phytochemistry 89, 71-77.
- Cuca, L.E., Martinez, J.C., Delle Monache, F., 1998. Alcaloides presentes em Hortia colombiana. Rev. Colomb. Quim. 27, 23-29.
- de Sousa, L.R.F., Ramalho, S.D., Burger, M.C., Nebo, D.M., Fernandes, L., da Silva, J.B., Iemma, M.F.G.F., Corrêa, M.R.C., Souza, C.J., Lima, D.H.F., Vieira, M.I.S.P.C., 2014. Isolation of arginase inhibitors from the bioactivity-guided fractionation of Byrsonima coccolobifolia leaves and stems. J. Nat. Prod. 77, 392-396.
- de Souza, V.A., da Silva, R., Pereira, A.C., Royo, V.A., Saraiva, J., Montanheiro, M., de Souza, G.H.B., da Silva Filho, A.A., Grando, M.D., Donate, P.M., Bastos, J.K., Albuquerque, S., Silva, M.L.A., 2005. Trypanocidal activity of (-)-cubebin derivatives against free amastigote forms of Trypanosoma cruzi Bioorg. Med. Chem. Lett. 15, 303-307.
- Ferreira, M.E., Arias, A.R., Yaluff, G., de Bilbao, N.V., Nakayama, H., Torres, S., Schinini, A., Guy, I., Heinzen, H., Fournet, A., 2010. Antileishmanial activity of furoquinolines and coumarins from Helietta apiculata Phytomedicine 17, 375-378.
- Fish, F., Waterman, P.G., 1973. Chemosystematics in the Rutaceae II. The chemosystematics of the Zanthoxylum/Fagara complex. Taxon 22, 177-203.
- Freitas, R.F., Prokopczyk, I.M., Zottis, A., Oliva, G., Andricopulo, A.D., Trevisan, M.T.S., Vilegas, W., Silva, M.G.V., Montanari, C.A., 2009. Discovery of novel Trypanosoma cruzi glyceraldehyde-3-phosphate dehydrogenase inhibitors. Bioorg. Med. Chem. 17, 2476-2482.
- Guíñez, R.F., Matos, M.J., Vazquez-Rodriguez, S., Santana, L., Uriarte, E., Olea-Azar, C., Maya, J.D., 2013. Synthesis and evaluation of antioxidant and trypanocidal properties of a selected series of coumarin derivatives. Future Med. Chem. 5, 1911-1922.
- Hohlemwerger, S.V.A., Sales, E.M., Costa, R.S., Velozo, E.S., Guedes, M.L.S., 2012. Alcaloides das cascas das raízes de Zanthoxylum spp.. Quim. Nova 35, 2173-2176.
- Iqbal, H., Ishfaq, M., Wahab, A., Abbas, M.N., Ahmad, I., Rehman, A., Zakir, M., 2016. Therapeutic modalities to combat leishmaniasis, a review. Asian Pac. J. Trop. Dis. 6, 1-5.
- Jain, S., Jacob, M., Walker, L., Tekwani, B., 2016. Screening North American plant extracts in vitro against Trypanosoma brucei for discovery of new antitrypanosomal drug leads. BMC Complement. Altern. Med., http://dx.doi.org/10.1186/s12906-016-1122-0
» http://dx.doi.org/10.1186/s12906-016-1122-0 - Kayser, O., Kiderlen, A.F., Croft, S.L., 2003. Natural products as antiparasitic drugs. Parasitol. Res. 90, S55-S62.
- Kutubi, M.S., Hashimoto, T., Kitamura, T., 2011. Improved synthesis of coumarins by iron(III)-catalyzed cascade reaction of propiolic acids and phenols. Synthesis, http://dx.doi.org/10.1055/s-0030-1258473
» http://dx.doi.org/10.1055/s-0030-1258473 - Leong, Y.-W., Harrison, L.J., Powell, A.D., 1999. Phenanthrene and other aromatic constituents of Bulbophyllum vaginatum Phytochemistry 50, 1237-1241.
- Maes, D., Riveiro, M.E., Shayo, C., Davio, C., Debenedetti, S., De Kimpe, N., 2008. Total synthesis of naturally occurring 5,6,7- and 5,7,8-trioxygenated coumarins. Tetrahedron 64, 4438-4443.
- Maes, D., Vervisch, S., Debenedetti, S., Davio, C., Mangelinckx, S., Giubellina, N., De Kimpe, N., 2005. Synthesis and structural revision of naturally occurring ayapin derivatives. Tetrahedron 61, 2505-2511.
- Mandlik, V., Patil, S., Bopanna, R., Basu, S., Singh, S., 2016. Biological activity of coumarin derivatives as anti-leishmanial agents. PLOS ONE 11, e0164585.
- Mesquita, M.L., Desrivot, J., Bories, C., Fournet, A., Paula, J.E., Grellier, P., Espindola, L.S., 2005. Antileishmanial and trypanocidal activity of Brazilian cerrado plants. Mem. Inst. Oswaldo Cruz 100, 783-787.
- Muzitano, M.F., Falcão, C.A.B., Cruz, E.A., Bergonzi, M.C., Bilia, A.R., Vincieri, F.F., Rossi-Bergmann, B., Costa, S.S., 2009. Oral metabolism and efficacy of Kalanchoe pinnata flavonoids in a murine model of cutaneous leishmaniasis. Planta Med. 75, 307-311.
- Nunes, F.M., Barros-Filho, B.A., de Oliveira, M.C.F., Andrade-Neto, M., de Mattos, M.C., Mafezoli, J., Pirani, J.R., 2005. 1H and 13C NMR spectra of 3,8-dimethoxyfuro[3,2-g]coumarin and maculine from Esenbeckia grandiflora Martius (Rutaceae). Magn. Reson. Chem. 43, 864-866.
- Östan, I., Saglam, H., Limoncu, M.E., Ertabaklar, H., Toz, S.Ö., Özbel, Y., Özbilgin, A., 2007. In vitro and in vivo activities of Haplophyllum myrtifolium against Leishmania tropica New Microbiol. 30, 439-445.
- Patiño, L.O.J., Prieto, R.J.A.L., Cuca, S.L.E., 2012. Zanthoxylum genus as potential source of bioactive compounds. In: Bioactive Compounds in Phytomedicine. InTech, P.I.R.E.
- Pusset, J., Lopez, J.L., Pais, M., Neirabeyeh, M.A., Veillon, J.-M., 1991. Isolation and 2D NMR studies of alkaloids from Comptonella sessilifoliola Planta Med. 57, 153-155.
- Randrianarivelojosia, M., Mulholland, D.A., Mc Farland, K., 2005. Prenylated coumarins from Cedrelopsis longibracteata (Ptaeroxylaceae). Biochem. Syst. Ecol. 33, 301-304.
- Reyes-Chilpa, R., Estrada-Muñiz, E., Vega-Avila, E., Abe, F., Kinjo, J., Hernández-Ortega, S., 2008. Trypanocidal constituents in plants: 7 Mammea-type coumarins. Mem. Inst. Oswaldo Cruz 103, 431-436.
- Paucar, R., Moreno-Viguri, Pérez-Silanez, E., 2016. Challenges in chagas disease drug discovery: a review. Curr. Med. Chem. 23, 3154-3170.
- Silva, C.V., Detoni, C.B., Velozo, E.S., Guedes, M.L.S., 2008. Alcalóides e outros metabólitos do caule e frutos de Zanthoxylum tingoassuiba A St. Hil.. Quim. Nova 31, 2071-2075.
- Tagboto, S., Townson, S., 2001. Antiparasitic properties of medicinal plants and other naturally occurring products. Adv. Parasitol. 50, 199-295.
- Tempone, A.G., Treiger Borborema, S.E., de Andrade, H.F., de Amorim Gualda, N.C., Yogi, Á., Salerno Carvalho, C., Bachiega, D., Lupo, F.N., Bonotto, S.V., Fischer, D.C.H., 2005. Antiprotozoal activity of Brazilian plant extracts from isoquinoline alkaloid-producing families. Phytomedicine 12, 382-390.
- WHO, 2012. Research Priorities for Chagas Disease, Human African Trypanosomiasis and Keishmaniasis. WHO Technical Report Series, 116.
- WHO, 2015a. Chagas disease in Latin America: an epidemiological update based on 2010 estimates. Wkly. Epidemiol. Record 6, 33-44.
- WHO, 2015b. Investing to Overcome the Global Impact of Neglected Tropical Diseases: Third WHO Report on Neglected Diseases 2015. WHO Document Production Services, Geneva, Switzerland.
- WHO, 2016. Leishmaniasis, Fact sheet, Updated September 2016. WHO Media Centre.
- Wink, M., 2012. Medicinal plants: a source of anti-parasitic secondary metabolites. Molecules 17, 12771-12791.
- Yoo, S., Kim, J., Kang, S., Son, K., Chang, H., Kim, H., Bae, K., Lee, C.-O., 2002. Constituents of the fruits and leaves of Euodia daniellii Arch. Pharm. Res. 25, 824-830.
Publication Dates
-
Publication in this collection
Sep-Oct 2018
History
-
Received
13 Sept 2017 -
Accepted
25 Apr 2018 -
Published
1 Aug 2018