Scorpion venom toxicity is of major concern due to its influence on human activities and public health. The cytotoxicity and apoptosis induced by scorpion L. quinquestriatus venom on two established eukaryotic cell lines (293T and C2C12) were analyzed. Both cultured cell lines were incubated with varying doses (10, 20, and 50 µg/ml) of scorpion venom in serum free medium (SFM) for 0.5, 1, 2, 4, and 8 hours at 37°C. The percentage of total lactate dehydrogenase (LDH) released in the culture during venom incubation was used as an index of cell damage. Control culture was treated with an equal amount of SFM. Cell injury was recognized morphologically and apoptosis was researched by a Fluorescing Apoptosis Detection System using the principle of TUNEL (TdT-mediated dUTP Nick-End Labelling) assay and confirmed by another assay concerning nuclear DNA staining with DAPI stain. Cytotoxicity was remarkable and cell survival highly reduced at the highest tested concentration (50 µg/ml). These effects were rapid and observed within 30 minutes. The apparent initial damage to the nucleus and lysis of the plasmalemma and/or organelle membranes, which was evident by a significant increase in cytosolic LDH release, suggested that this toxin acts at the membrane level. The morphological changes that occurred in apoptotic cells include condensation and compartmentalization of nuclear and cytoplasmic materials into structurally preserved membrane-bound fragments or blebs. The cytotoxic effects are dose and time dependent and cell death by apoptosis was more characteristic of 293T cells than C2C12 cells. The apoptotic effects were more prominent and clear in the early stages of toxicity, while other forms of cell damage such as swelling, rupture, and/or necrosis occurred at later stages.
scorpion venom; Leiurus quinquestriatus; cytotoxicity; apoptosis; LDH
ORIGINAL PAPER
Cytotoxic and apoptotic effects of scorpion Leiurus quinquestriatus venom on 293T and C2C12 eukaryotic cell lines
M. A. A. Omran
Zoology Department, Faculty of Science, Suez Canal University, Ismailia, Egypt
Correspondence Correspondence to M. A. A. Omran Zoology Department, Faculty of Science, Suez Canal University Ismailia, Egypt maomran@ismailia.ie-eg.com
ABSTRACT
Scorpion venom toxicity is of major concern due to its influence on human activities and public health. The cytotoxicity and apoptosis induced by scorpion L. quinquestriatus venom on two established eukaryotic cell lines (293T and C2C12) were analyzed. Both cultured cell lines were incubated with varying doses (10, 20, and 50 µg/ml) of scorpion venom in serum free medium (SFM) for 0.5, 1, 2, 4, and 8 hours at 37°C. The percentage of total lactate dehydrogenase (LDH) released in the culture during venom incubation was used as an index of cell damage. Control culture was treated with an equal amount of SFM. Cell injury was recognized morphologically and apoptosis was researched by a Fluorescing Apoptosis Detection System using the principle of TUNEL (TdT-mediated dUTP Nick-End Labelling) assay and confirmed by another assay concerning nuclear DNA staining with DAPI stain. Cytotoxicity was remarkable and cell survival highly reduced at the highest tested concentration (50 µg/ml). These effects were rapid and observed within 30 minutes. The apparent initial damage to the nucleus and lysis of the plasmalemma and/or organelle membranes, which was evident by a significant increase in cytosolic LDH release, suggested that this toxin acts at the membrane level. The morphological changes that occurred in apoptotic cells include condensation and compartmentalization of nuclear and cytoplasmic materials into structurally preserved membrane-bound fragments or blebs. The cytotoxic effects are dose and time dependent and cell death by apoptosis was more characteristic of 293T cells than C2C12 cells. The apoptotic effects were more prominent and clear in the early stages of toxicity, while other forms of cell damage such as swelling, rupture, and/or necrosis occurred at later stages.
Keywords: scorpion venom, Leiurus quinquestriatus, cytotoxicity, apoptosis, LDH.
INTRODUCTION
Scorpion venoms are the subject of many investigations. Most of these studies deal with the toxic effects of venom and its components, whilst others are concerned with the characterization and identification of different constituents in this complex protein substance (22,27,30,36). Several studies have suggested using some animal venoms and venom components as tools to resolve particular problems in their own research and also to explain the mode of action of specific ion channels (15,23,25,26,48).
Scorpions are arachnids of wide geographical distribution (particularly in tropical and subtropical areas of the world) and possess a venom apparatus composed of a pair of glands and a sting. Venoms contain small molecular weight peptides capable of causing cell function impairment by interfering with ion channel permeability of excitable cell membranes (2,12). Scorpion venoms consist of a mixture of many pharmacologically active proteins, and they have higher toxin contents than their snake counterparts. Most scorpion toxins contain four disulphide bridges, the location of which is quite different from that found in snake toxins. These differences may in part explain the diverse modes of neurotoxic action of venoms from these species (40).
The species most responsible for severe human envenoming belongs to the Buthidae family. The Buthid scorpion Leiurus quinquestriatus is found throughout Egypt, Israel, Syria, Jordan, and Saudi Arabia (17). It is one of the commonest and most dangerous species in these areas. Injection of just 17 mg of its venom into an average sized human adult results in a less than 50% chance of survival (1). In mice, LD50 is 0.25 µg/g (40). Severe envenoming may develop rapidly from the first sign symptoms with progression often taking only 5 to 30 minutes. Severe systemic complications such as heart failure, pulmonary edema, convulsions, and severe histopathological changes, including degeneration of cardiac fibers, focal necrosis, interstitial edema and hemorrhage, and coma worsen the prognosis (9,28,29,31,32,39).
Cell death has been considered a degenerative phenomenon, a running down of the metabolic activity of the cell (8). Most cells from higher eukaryotes have the ability to self-destruct by activation of an intrinsic cellular suicide program when they are no longer needed or have become seriously damaged by internal or external stimuli. This normal physiological process, known as programmed cell death (apoptosis), refers to morphological alterations exhibited by dying cells that include shrinkage, membrane blabbing, chromatin condensation, and fragmentation. Cells undergoing apoptosis often fragment into membrane-bound apoptotic bodies that are readily phagocytized and digested by macrophages or neighboring cells without generating an inflammatory response (7). These changes distinguish apoptosis from cell death by necrosis. Necrosis refers to the morphology most often seen when cells die from severe and sudden injury, such as ischemia. In necrosis, there are early changes in mitochondrial shape and function, the cell loses its ability to regulate osmotic pressure and consequently swells and ruptures. Cell contents are spilled into the surrounding tissue, resulting in the generation of a local inflammatory response (13).
Most data on the mechanism of toxin action from venomous animals were obtained by in vitro experiments using several different cell lines. As there is similarity between necrosis and apoptosis, it is critical to differentiate between them. This paper aims to provide a quantitative and qualitative characterization of biological activities of L. quinquestriatus venom on two established eukaryotic cell lines (293T and C2C12) in order to gain a better understanding of the cytotoxic, apoptotic, and/or necrotic effects of this lethal toxin.
MATERIALS AND METHODS
Venom collection:L. quinquestriatus scorpions were captured from Southern Egypt around the deserts of Nasser Lake in Aswan. In this area, L. quinquestriatus occurs in relatively dense populations and is a persistent threat to the local community. Captive scorpions were kept alive in separate containers and venom collected according to the method described by Omran and McVean (30).
Cell lines: The 293T and C2C12 cell lines were used in this study. The 293T cell line (293 with SV40 virus large T antigen) is a permanent line of primary human embryonic kidney cells, which has favorable tissue culture, transfection, DNA replication, gene expression, and protein production properties (43). The C2C12 cell line is a sub-clone derived from a mouse myoblast cell line from normal adult C3H leg muscle. The cell line differentiates rapidly and produces extensive contracting myotubes expressing characteristic muscle proteins. It also provides an excellent model to study myogensis and cell differentiation in vitro (44). Both cell lines were propagated in DMEM medium (Sigma, St. Louis, MO, USA) supplemented with 10% fetal bovine serum (FBS), 2mM L-glutamine, and the antibiotics penicillin (10,000-units/L) and streptomycin (100-mg/L). The cell lines were incubated in flasks as monolayers and cultured at 37°C / 8% CO2 in a fully humidified atmosphere.
Quantification of venom cytotoxicity: Cells used in this study were harvested by trypsination (0.25% trypsin in PBS), resuspended in fresh medium, and plated in 24-well plates at 1.5 x 104 2 x 105 cells/well. Incubation conditions were as above. After 24 hours, the cultures were washed with serum free medium (SFM) and various concentrations (10, 20, and 50 µg/ml SFM) of venom were added and the cells were incubated for 8 h. (SFM was used to avoid the possible interaction between venom and FBS).
Toxicity was assayed by measuring the activity of the cytosolic enzyme lactate dehydrogenase (LDH) released into the culture medium after membrane rupture (11). Samples from clarified medium of treated and untreated control wells (6 replica/treatment) were taken after intervals of 0.5, 1, 2, 4, and 8 h of incubation and the LDH activity measured using the cytotoxicity assay CytoTox 96® (Promega, Madison, WI, USA) in conjunction with a fully automated microplate reader photometer (HTIII, Anths Labtec Instruments, Salzburg). This assay system for quantifying LDH release from cell can detect whether cell and apoptotic bodies remain enclosed by intact plasma membrane, and thus, can be used to discriminate between apoptosis and necrosis (24).
Cell damage and morphological changes to both cell lines were investigated using a light phase contrast microscope (Leica DMIRB, Germany) and photos were taken at each time point by computerized Colour Coolview camera using the Image-Pro® Lite software (Media Cybernetics, Silver Spring, MD, USA).
Venom stability in culture: To examine variations of venom activity in the liquid medium, culture medium containing scorpion venom at the lowest concentration used in this study (10 µg/ml) were incubated at 37°C/8% CO2 in a fully humidified atmosphere. After 24-h incubation, samples of venom solution were tested on the 293T cells by CytoTox 96® assay.
Apoptosis assays: A morphological analysis of apoptosis was carried out to differentiate between the necrotic and apoptotic effects of the scorpion venom used in this study. Both the C2C12 and 293T cells were grown on lab-Tek chamber slides and treated with the same doses of scorpion venom (10, 20, and 50 µg/ml SFM) under the same culture conditions described above. Slides were incubated for 1, 2, 4, and 8 h. After treatment, slides were washed twice with PBS and processed directly in the apoptosis detection assays. The first assay used was a Fluorescein Apoptosis Detection System (Promega Madison, WI, USA), which is designed for specific detection and quantification of apoptotic cells within a cell population consisting of both apoptotic and non-apoptotic cells. This system provides simple, rapid, and accurate detection of apoptotic cells in situ at the single cell level or in cell suspensions (24). It measures the fragmented DNA of apoptotic cells by catalytically incorporating fluorescein-12-dUTP at the 3-hydroxyl ends of the fragmented DNA using the enzyme Terminal deoxynucleotidyl Transferase (TdT), which forms a polymeric tail using the principle of TUNEL (TdT-mediated dUTP Nick-End Labelling) assay. According to this protocol, slides were washed and counterstained with 1 µg/ml propidium iodide for 15 minutes as a final step. Slides were mounted and photographed with a Leica DMIRB fluorescent microscope and dual exposure micrographs recorded at 400X magnification using standard fluorescing filter sets. Apoptotic cells have incorporated fluorescein-12-dUTP (yellow-green at 520±20 nm), indicating the presence of DNA fragments. Propidium iodide (red at 620 nm) was used as a counter-stain for all cells. This system is useful because morphological observation and verification of the apoptotic phenotype can be done simultaneously to rule out necrosis (13,37).
To confirm the occurrence of apoptosis, another stain was used, 4,6-diamidino-2-phenylindole dihydrochloride hydrate (DAPI) (Sigma, USA). DAPI binds to double stranded DNA by forming a stable complex, which fluoresces about 20 times more than DAPI alone, and the fluorescence is unchanged over a wide pH range. The complex is stable for hours at room temperature and is not photo-dissociated during assay (16,37). Cell preparation and treatment were done as described above. Slides were stained with DAPI (dilution 1:1000 in PBS) for 10 minutes in the dark at 0-5ºC.
Slides were examined and photographed with a Leica DMIRB fluorescent microscope at 400X magnification, using standard fluorescing filter sets. Apoptosis reveals several characteristic morphological changes such as chromatin condensation, nuclear fragmentation, and cytoplasm membrane disorientation where the cytoplasm fluoresces blue and the nucleus pink to red (7,38).
Statistical analysis: Data were analyzed by one way ANOVA and statistical probability of p < 0.05 was considered significant.
RESULTS
Detection of LDH activity
Results obtained from the venom stability test revealed that scorpion venom is stable during the incubation time period (24 h) with the culture medium, and its cytotoxic activities on the selected cell lines were highly significant.
Lactate dehydrogenase release from cultured cells exposed to a biomaterial provides a sensitive and accurate marker for cellular toxicity. Scorpion venom induced identical dose-response effect on 293T cells (Figure 1), releasing 30, 48, and 76% of LDH activity after 8 hours at concentrations of 10, 20, and 50 µg/ml, respectively. This effect was virtually insignificant at 10 µg/ml dose and the only significant change at 20 µg/ml dose compared to control levels was recorded after 8 hours. The highest treatment (50 µg/ml) used in this study induced significant increase in LDH levels throughout the whole period reaching a plateau after 4 hours of incubation. Also, the highest scorpion dose released significant amounts of intracellular LDH after 4 and 8 hours of treatment compared to the other two doses (10 and 20 µg/ml).
Cytotoxic effect of L. quinquestriatus venom on the 293T cell line. Cultured permanent cell line (293T) of primary human embryonic kidney was incubated with varying doses (10, 20, and 50 µg/ml) of scorpion venom in serum free medium (SFM) for 0.5, 1, 2, 4, and 8 hours at 37°C. The percentage of total LDH released in the culture during venom incubation was used as an index of cell damage induced by the toxin. Control culture was treated with an equal amount of SFM. Values are means ± S.E. of 6 replica/treatment. Data were analyzed by one way ANOVA and statistical probability of p < 0.05 was considered significant:
To extend the analysis of in vitro cytotoxicity, similar experiments were performed with the cultured mouse myoblast cell line C2C12. Concentrations of 10 and 20 µg/ml scorpion venom induced mild damage to the C2C12 cell line compared to control values (Figure 2), and the only significant elevation in LDH release (36%) was recorded after 8 hours of incubation with 20 µg/ml dose. At a dose of 50 µg/ml, a significant increase was observed at all time points compared to control levels, whereas 55% of LDH was detected in the culture media at the end of the incubation period (8 hours). This value was also significant compared to LDH levels at 10 µg/ml dose at the same time point. It is important to mention that in vitro LDH release in 293T cells is higher than the C2C12 cell line, indicating that the cytotoxic effects of L. quinquestriatus venom are more potent to the 293T cell line.
Cytotoxic effect of L. quinquestriatus venom on the C2C12 cell line. Cultured fibroblasts from the C2C12 cell line were incubated with varying doses (10, 20, and 50 µg/ml) of scorpion venom in serum free medium (SFM) for 0.5, 1, 2, 4, and 8 hours at 37°C. The percentage of total LDH released in the culture during venom incubation was used as an index of cell damage induced by the toxin. Control culture was treated with an equal amount of SFM. Values are means ± S.E. of 6 replica/treatment. Data were analyzed by one way ANOVA and statistical probability of p < 0.05 was considered significant:
Microscopic investigations of venom toxicity
The morphological changes induced by L. quinquestriatus venom on 293T cells are illustrated in Figure 3. Various structural abnormalities were recorded in 293T cells as a result of incubation with different concentrations of scorpion toxin. The 293T cells in the cultured media of control samples were homogeneously distributed in the culture field. They exhibited polygonal shape with distinct boundaries and homogenous or slightly granulated cellular contents (Figure 3A). Cells incubated with 10 µg/ml dose (Figure 3B) were more or less similar in morphological appearance to those of the control cells except for the occurrence of some cellular swelling and presence of a few empty looking areas (vacuoles-like) devoid of any cultured cells. Cellular swelling was increased after incubation with 20 µg/ml venom (Figure 3C), and the density of cellular contents was also increased. Some cells were ruptured and lost the continuity of their surrounding membranes, and the numbers of empty-looking areas were also increased. Cells incubated with the highest dose (50 µg/ml) of scorpion venom (Figure 3D) had observed similar changes with more severity.
Figure 3. Light micrographs (phase contrast) of cultured permanent cell line (293T) of primary human embryonic kidney after 8 hours of incubation with varying doses (10, 20, and 50 µg/ml) of L. quinquestriatus venom in serum free medium (SFM) at 37°C (X 400).
A: Untreated control cells were homogeneously distributed in the culture field. The cells exhibited polygonal shape with distinct boundaries and homogenous or slightly granulated cellular contents.
B: Cells incubated with 10 µg/ml dose. Note some cellular swelling (black arrows) and a few vacuole-like areas (white arrows, V) devoid of any cultured cells.
C: Cellular swelling was increased after incubation with 20 µg/ml venom and the density of the cellular contents was also increased. Some cells were ruptured (D) and lost the continuity of their surrounding membranes and the numbers of vacuoles were also increased.
D: Cells incubated with the highest dose (50 µg/ml) of venom showed similar changes with more severity.
The in vitro biological activity of scorpion venom was tested in tissue culture preparation of another cell type, the mouse myoblast cell line C2C12. Untreated cells showed the characteristic appearance of the common fibroblasts (Figure 4A). The C2C12 cells were thin and elongated with two tapering ends. Scorpion venom induced less cytotoxic and morphological alterations to C2C12 cell line compared to the 293T cell line. Most C2C12 cells retained their common characteristic structure after incubation with the smallest tested dose (10 µg/ml). However, few cells exhibited rounded appearance rather than the normal elongated configuration (Figure 4B). Although the same effects were observed at 20 µg/ml dose, more cells showed rounded shape with density accumulated contents and few empty looking areas were clear in the culture media (Figure 4C). These vacuolated areas reveal that some cells have disappeared, which might be related to cell damage and/or cell necrosis caused by venom toxic components. After incubation with 50 µg/ml dose, most cells became roughly oval or irregular in shape with highly condensed contents; very few cells still retained their fibroblastic common appearance (Figure 4D). A remarkable increase in the spontaneous muscle contraction was recorded especially after treatment with the highest dose. It is worth emphasizing that there were fewer vacuolated areas in the C2C12 cells compared to the 293T cell line at the same dose concentrations.
Figure 4. Light micrographs (phase contrast) of the mouse myoblast cell line C2C12 after 8 hours of incubation with varying doses (10, 20, and 50 µg/ml) of L. quinquestriatus venom in serum free medium (SFM) at 37°C (X 400).
A: Long untreated control myotubes crossing the field and the cell membrane is smooth and without irregularities. Some fibroblasts are present.
B: Cells incubated with 10 µg/ml dose. Note the presence of a few vacuole-like areas (V), and a few numbers of cells exhibited rounded and oval appearance rather than the normal elongated configuration (white arrows).
C: Cells incubated with 20 µg/ml dose, more cells showed rounded and oval shapes with dense accumulated contents. A few empty looking areas were clear in the culture.
D: Cells incubated with the highest dose (50 µg/ml) of venom. Most cells became roughly oval or irregular in shape with highly condensed contents and very few cells still retained their fibroblastic common appearance. Extensive areas of the myotubes are vacuolated.
Apoptosis assays
The first assay used in this study was the fluorescing apoptosis detection system. This technique measures the fragmented DNA of the apoptotic cell. DNA fragments are labeled green fluorescence within cells undergoing apoptosis. Control cells showed the red fluorescent propidium iodide staining after 2, 4, and 8 hours of incubation in SFM without any green labeled cells (Figure 5 - 1A, 1B, and 1C). Fluorescence green labeled cells intermixed with red labeled cells were observed after incubation with 10 µg/ml scorpion venom (Figure 5 - 2A, 2B, and 2C) and the number of apoptotic cells (green) were less than non-apoptotic cells (red). Dose dependent increases in number of apoptotic cells were recorded after 2 and 4 hours as a result of treating cells with 20 (Figure 5 3A and 3B) and 50 µg/ml venom (Figure 5 4A and 4B). The overall number of recognized cells, apoptotic and non-apoptotic, were markedly decreased after 8 hours (Figure 5 - 4C) of incubation with the highest venom dose (50 µg/ml). Total cell number reduction might be related to the complete destruction and loss of some treated cells either by necrosis and/or cell rupture and damage caused by high venom doses.
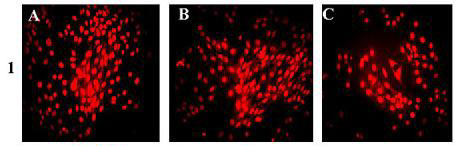
Figure 5. Representative photomicrographs showing labeling of DNA strand breaks associated with apoptosis by TUNEL assay using the Apoptosis Detection system, Fluorescein, in the presence of TdT enzyme. The C2C12 cell line was grown on lab-Tek chamber slides and treated with different doses (10, 20, and 50 µg/ml) of L. quinquestriatus venom. Slides were incubated for 1, 2, 4 and 8 hours in serum free medium (SFM) at 37°C. After treatment, slides were washed twice with PBS and analyzed as indicated in the text. Note the DNA fragmentation, as evidenced by Fluorescein -12-dUTP incorporation (yellow-green fluorescence), indicative of apoptosis (Figure 5 - 2, 3, and 4). Figure 5 1 shows the red fluorescent of propidium iodide staining nonapoptotic cells (X 400).
1= Control untreated cells;
2= Cells incubated with 10 µg/ml of L. quinquestriatus venom.
3= Cells incubated with 20 µg/ml of L. quinquestriatus venom.
4= Cells incubated with 50 µg/ml of L. quinquestriatus venom.
A= After 2 hours; B= After 4 hours; C= After 8 hours.
Results obtained from the second technique illustrated that nuclear DNA staining of both cell lines, 293T and C2C12, with DAPI stain showed evidence of chromatin condensation and DNA fragmentation, which are generally considered to be characteristic of apoptosis. Cells incubated with SFM (control) showed a dominance of fluorescent blue color of normal non-apoptotic cells (Figure 6-1). Appearance of DAPI-positive nuclei (fluorescent red) was found beginning at 2 and 4 hours after incubation with 10 and 20 µg/ml scorpion venom and as early as 1 and 2 hours after treatment with 50 µg/ml dose (Figure 6). Apoptosis, as detected by DAPI staining, was significantly higher in cells treated with the highest venom dose (Figure 6-4) compared to the number of apoptotic cells observed after incubation with lower doses, 10 and 20 µg/ml, as shown in Figure 6 - 2 and 3, respectively. It is important to mention that both assays used to detect apoptosis revealed that the 293T cells have higher tendency to undergo apoptosis than the C2C12 as a result of treatment with L. quinquestriatus venom.
Figure 6. Representative photomicrographs showing DAPI staining of the 293T cells after 4 hours of incubation with L. quinquestriatus venom. Cell preparation and treatment were done as described in Figure 5. Slides were stained with DAPI (dilution 1:1000 in PBS) for 10 minutes in the dark at 0-5°C. Apoptosis reveals several characteristic morphological changes such as chromatin condensation and nuclear fragmentation (arrows) where the cytoplasm fluoresces blue and the nucleus pink to red (X 400).
1= Control untreated cells;
2= Cells incubated with 10 µg/ml of L. quinquestriatus venom.
3= Cells incubated with 20 µg/ml of L. quinquestriatus venom.
4= Cells incubated with 50 µg/ml of L. quinquestriatus venom.
DISCUSSION
Despite the great advances in understanding the morphological and biochemical alterations associated with substances and chemicals induced cell injury, it has to be realized that the knowledge acquired is still insufficient to establish which of changes lead to cell death and which are secondary disturbances. In 1982, Farber (8) suggested that under some conditions cell death might not be a passive event but may rather be the result of a more active process, like the overproduction of some enzyme or other protein, cell suicide instead of cell homicide. Apoptosis, programmed cell death, involves a cascade of cytoplasmic and nuclear events that result in a series of morphological changes and eventually cause cell demise (14). It can be initiated by a variety of different stimuli that lead to a convergence of biochemical signaling pathways into a common collection of executioner molecules (24). On the other hand, necrosis is the death of cells or tissue as a result of external trauma such as physical damage or lack of oxygen. It results in cell lysis and extensive damage to surrounding tissues (35). This study attempts to clarify some of the cytotoxic effects of L. quinquestriatus venom and to prove that apoptosis occurs on cultured cell lines induced by this natural toxin using some recent in vitro techniques.
This investigation shows that the damage induced to both cell lines by scorpion venom is direct and is not secondary to disruption of the microcirculation, which causes muscle necrosis in animals and humans (3). The cytotoxicity was remarkable and cell survival was highly reduced at the highest tested concentration (50 µg/ml). Because of the rapid effects observed within 30 minutes and lysis of the plasmalemma and/or organelle membranes that immediately caused the greatest level of cell population death, as seen by significant increase in the cytosolic LDH release, we suggest that this toxin acts at the membrane level. Numerous previous investigations (4,6,21) have reported the same loci of action for some other natural toxins. Furthermore, Bruses et al. (6) suggested that some myotoxins either cross the sarcolemma by endocytosis, or are translocated by a carrier, or move through pores or channels in the membranes. This allows the passage of ions down their concentration gradient, resulting in osmotic changes in organelles, followed by several unidentified mechanisms leading to cell death. This might be one of the reasons that produces apoptosis (cell suicide) for both cell lines as a result of treatment with scorpion venom. In this respect, Lyonel and Esther (18) reported that the death signal and apoptosis could be set in motion by diverse stimuli such as chemical toxicity, radiation, or genotoxic damage.
Some of the histological images of dying C2C12 cells recorded in this investigation after treatment with the highest venom dose are similar to the necrosis that pathologists have described in muscles, which is common to a wide variety of pathological and toxicological conditions. In all these cases, it has been hypothesized that dilation of the organelles is related to an accumulation of fluid in the sarcoplasmic reticulum and T-tubules (6). It was reported that some scorpion venoms have a very profound necrotic effect in vivo (33,39,40,46).
The morphological changes that occurred in apoptotic cells include condensation and compartmentalization of nuclear and cytoplasmic materials into structurally preserved membrane-bound fragments or blebs. Cell death by apoptosis was more characteristic to the 293T cells than the C2C12 cells. The apoptotic mechanism induced by scorpion venom can be explained by the findings of McConkey and Orrenius (19,20), Parekh and Penner (34), and Sylivie, et al. (38). They have reported that the rise in intracellular free calcium triggers the apoptotic cascade in a large number of in vitro models. This calcium rise can result either from influx from the extracellular space, mobilization of calcium intracellular stores (endoplasmic reticulum, mitochondria), or from both through the process of calcium-induced calcium release (5). Yarom and Braun (45,46) and Fayet et al. (10) reported that scorpion venom elevated the calcium content in the myocardiac fibers of experimental animals. Recent studies (26,41,42,47) confirmed these findings. They concluded that the crude venom of two African scorpion species and some of their purified fractions evoked a release of calcium from intracellular calcium stores and also activate calcium release channels incorporated into planar lipid bilayers.
Several conclusions can be reached from this study. It is clear that the cytotoxic effects are dose and time dependent, and although L. quinquestriatus venom kills cells by different mechanisms, the relationship between these mechanisms is unclear. The apoptotic effects are more prominent and clear in the early stages of toxicity, while other forms of cell damage such as swelling, rupture, and/or necrosis occurred in the later stages. It was indeed unexpected that L. quinquestriatus venom induced an early significant apoptotic effect rather than necrosis on both cell lines; however, the severity of this effect is considerably different, high on the 293T cell line and moderate on the C2C12 cell line. This might reflect a greater selectivity of scorpion venom for primary human embryonic kidney cells than for the other type of tested cell line. Also, this effect might be mediated through specific unknown receptor(s) located on the plasma membrane or in the cytosol, affecting the cell signaling system leading to cell death. This postulation is in part in agreement with the reports of Bruses et al. (6). They mentioned the possibility that some toxins must bind to a specific receptor in the membrane before they can exert their action. If such a toxin receptor is extensively present on certain membranes, such as 293T cells, this could explain the high selectivity of scorpion toxins for these cells. This hypothesis, although attractive, awaits experimental confirmation.
ACKNOWLEDGEMENTS
The author thanks Prof. George Dickson, Centre for Biomedical Sciences, School of Biological Sciences, Royal Holloway, University of London, UK for using his laboratory facilities, and Prof. Tarek R. Rahmy, Zoology Department, Faculty of Science, Suez Canal University, Ismailia, Egypt for his valuable comments.
Received July 24, 2002
Accepted November 11, 2002
References
- 1 ANDERSON RC. Scorpions: the ancient arachnids. Idaho Museum of Natural History. Spec. Publ., 1983, 8, 1-21.
- 2 ANDERSON PAV., GREENBERG RM. Phylogeny of ion channels: clues to structure and function. Comp. Biochem. Physiol. B., 2001, 129, 17-28.
- 3 ARCE V., BRENES F., GUTIERREZ JM. Degenerative and regenerative changes in murine skeletal muscle after injection of venom from the snake Bothrops asper: a histochemical and immunocytochemical study. Int. J. Exp. Pathol., 1991, 72, 211-26.
- 4 BALBONI F., BERNABEI PA., SANNA A., ROSSI FP., DELFINO G. Cutaneous venom of Bombina variegata pachypus (Amphibia, Anura): effects on the growth of the human HL60 cell line. Cell Biol. Int. Rep., 1992, 16, 329-38.
- 5 BERRIDGE JM., BOOTMAN MD., LIPP P. Calcium-a life and death signal. Nature, 1998, 395, 645-8.
- 6 BRUSES JL., CAPASO J., KATZ E., PILAR G. Specific in vitro biological activity of snake venom myotoxins. J. Neurochem., 1993, 60, 1030-42.
- 7 ESSBAUER S., AHNE W. The epizootic haematopoietic necrosis virus (Iridoviridae) induces apoptosis in vitro J. Vet. Med. B. Infect. Dis. Vet. Public Health, 2002, 49, 25-30.
- 8 FARBER E. Chemical carcinogenesis: a biological perspective. Am. J. Pathol., 1982, 106, 271-96.
- 9 FATANI AJ., FURMAN BL., ZEITLIN IJ. The involvement of plasma kinins in the cardiovascular effects of Leiurus quinquestriatus scorpion venom in anaesthetised rabbits. Toxicon, 1998, 36, 523-36.
- 10 FAYET G., COURAUD F., MIRANDS F., LISSITZKY S. Electro-optical system for monitoring activity of heart cells in culture: application to the study of several drugs and scorpion toxins. Eur. J. Pharmacol., 1974, 27, 165-74.
- 11 FERBER E. Biochemical pathology. Annu. Rev. Pharmacol., 1971, 11, 71-96.
- 12 GORDON D., SAVARIN P., GUREVITZ M., ZINN-JUSTIN S. Functional anatomy of scorpion toxins affecting sodium channels. J. Toxicol. Toxin Rev., 1998, 17, 131-59.
- 13 HINES MD., ALLEN-HOFFMANN L. Analysis of DNA fragmentation in epidermal keratinocytes using the apoptosis detection system, fluorescein. Promega notes, 1996, 59, 30-7.
- 14 HOUCHINS JP. Immunotoxin-induced apoptosis. Stem Cells, 2000, 18, 348-85.
- 15 HUGHES AL. Evolutionary diversification of the mammalian defensins. Cell. Mol. Sci., 1999, 56, 94-103.
- 16 KHANOBDEE K., SOOWANNAYAN C., FLEGEL TW., UBOL S., WITHYACHUMNARNKUL B. Evidence for apoptosis correlated with mortality in the giant black tiger shrimp Penaeus monodon infected with yellow head virus. Dis. Aquat. Organ., 2002, 48, 79-90
- 17 LUCAS SM., MEIER J. Biology and distribution of scorpions of medical importance. In: MAYR E. Animal species and evolution. Massachusetts: Harvard University Press, 1995: 205-19
- 18 LYONEL GI., ESTHER DI. Apoptosis. Stem cells, 1999, 17, 306-13.
- 19 MCCONKEY DJ., ORRENIUS S. The role of calcium in the regulation of apoptosis. J. Leukoc. Biol., 1996a, 59, 775-83.
- 20 MCCONKEY DJ., ORRENIUS S. Signal transduction pathway in apoptosis. Stem Cells, 1996b, 14, 619-31.
- 21 MEBS D., OWNBY CL. Myotoxic components of snake venoms: their biochemical and bilogical activities. Pharmacol. Ther., 1990, 48, 223-36.
- 22 MEIER J., STOKER K. On the significance of animal experiments in toxinology. Toxicon, 1989, 27, 91-104.
- 23 MILLER C. The charybdotoxin family of K+ channel-blocking peptides. Neuron, 1995, 15, 5-10.
- 24 MORAVEC R., RISS T. Assay system for detecting apoptosis and cell death. Promega notes, 1998, 68, 13-8.
- 25 MOSKOWITZ H., HERRMAN R., JAMES AD., HAMMOCK BD. A depressant insect-selective toxin analog from the venom of the scorpion Leiurus quinquestriatus hebraeus: purification and structure/function characterization. Eur. J. Biochem., 1998, 254, 44-9.
- 26 NABHANI T., ZHU X., SIMEONI I., SORRENTINO V., VALDIVIA HH., GARCIA J. Imperatoxin a enhances Ca2+ release in developing skeletal muscle containing ryanodine receptor type 3. Biophys. J., 2002, 82, 1319-28.
- 27 OMRAN MAA. Are the toxic effects of scorpion Leiurus quinqestriatus venom on rat's cardiovascular system related to the route of administration? J. Egypt. Ger. Soc. Zool., 1996, 20, 29-54.
- 28 OMRAN MAA., ABDEL-RAHMAN MS. Effect of scorpion Leiurus quinquestriatus (H&E) venom on the clinical Chemistry Parameters of the rat. Toxicol. Lett., 1992, 61, 99-109.
- 29 OMRAN MAA., ABDEL-RAHMAN MS. Effect of scorpion venom on in vitro rat blood glutathione levels and erythrocyte osmotic fragility. J. Nat. Toxins, 1994, 3, 69-78.
- 30 OMRAN MAA., MCVEAN A. Intraspecific variation in scorpion Leiurus quinquestriatus venom collected from Egypt (Sinai and Aswan deserts). J. Toxicol. Toxin Rev., 2000, 19, 247-64.
- 31 OMRAN MAA., ABDEL-RAHMAN MS., NABIL ZI. Effect of scorpion Leiurus quinquestriatus (H&E) venom on rat's heart rate and blood pressure. Toxicol. Lett., 1992a, 61, 111-21.
- 32 OMRAN MAA., ABDEL-RAHMAN MS., NABIL ZI. The role of atropine and propranolol in mitigating the toxic effects of scorpion venom on rat electrocardiogram. Toxicol. Lett., 1992b, 61, 175-84.
- 33 OMRAN MAA., NABIL ZI., IBRAHIM HA. ECG abnormalities induced by scorpion venom administration, the effect and the mechanism. Qatar Univ. Sci. J., 1994, 14, 351-9.
- 34 PAREKH AB., PENNER R. Store depletion and calcium influx. Physiol. Rev., 1997, 77, 901-30.
- 35 ROBBINS SL., ANGELL M. Basic Pathology. London: Saunders Company, 1976. 705p.
- 36 SAUTIERE P., CESTELE S., KOPEYAN C., MARTINAGE A., DROBECQ H., DOLJANSKY Y., GORDON D. New toxins acting on sodium channels from the scorpion Leiurus quinquestriatus hebraeus suggest a clue to mammalian vs. Insect selectivity. Toxicon, 1998, 36, 1141-54.
- 37 SHIMIZU A., MASUDA Y., KITAMURA H., ISHIZAKI M., OHASHI R., SUGISAKI Y., YAMANAKA N. Complement-mediated killing of mesangial cells in experimental glomerulonephritis: cell death by a combination of apoptosis and necrosis. Nephron, 2000, 86, 152-60.
- 38 SYLVIE M., LEYBAERT L., KATHARINA D. First and second messenger role of calcium: survival versus apoptosis in serum-free cultured granulosa explants. Ann. N. Y. Acad. Sci., 2000, 926, 101-15
- 39 TARASIUK A., KHVATSKIN S., SOFER S. Effects of antivenom serotherapy on hemodynamic pathophysiology in dogs injected with of Leiurus quinquestriatus scorpion venom. Toxicon, 1998, 36, 963-71.
- 40 TU AT. Venoms: chemistry and molecular biology New York: Wiley, 1977. 560p.
- 41 VALDIVIA HH., FUENTES O., EL-HAYEK R., MORRISSETTE J., CORONADO R. Activation of the ryanodine receptor Ca2+ release channel of sarcoplasmic reticulum by a novel scorpion venom. J. Biol. Chem., 1991, 266, 19135-8.
- 42 VALDIVIA HH., KIRBY MS., LEDERER WJ., CORONADO R. Scorpion toxins targeted against the sarcoplasmic reticulum Ca(2+)-release channel of skeletal and cardiac muscle. Proc. Natl. Acad. Sci., 1992, 89, 12185-9.
- 43 WAHLFORS JJ., ZULLO SA., LOIMAS S., NELSON DM., MORGAN RA. Evaluation of recombinant alphaviruses as vectors in gene therapy. Gene ther., 2000, 7, 472-80.
- 44 YAFFE D., SAXEL O. Serial passaging and differentiation of myogenic cells isolated from dystrophic mouse muscle. Nature, 1977, 270, 725-7.
- 45 YAROM R., BRAUN K. Myocardial pathology following scorpion venom injection. Isr. J. Med. Sci., 1969, 5, 849-52.
- 46 YAROM R., BRAUN K. Ca2+ changes in the myocardium following scorpion venom injections. J. Mol. Cell. Cardiol., 1971, 2, 177-9.
- 47 ZAMUDIO FZ., GURROLA GB., AREVALO C., SREEKUMAR R., WALKER JW., VALDIVIA HH., POSSANI LD. Primary structure and synthesis of Imperatoxin A (IpTx(a)), a peptide activator of Ca2+ release channels/ryanodine receptors. FEBS Lett., 1997, 405, 385-9.
- 48 ZILBERBERGN., GORDON D., PELHATE M., ADAMS ME., NORRIS TM., ZLOTKIN E., GUREVITZ M. Functional expression and genetic alteration of an alpha scorpion neurotoxin. Biochemistry, 1996, 35, 10215-22.